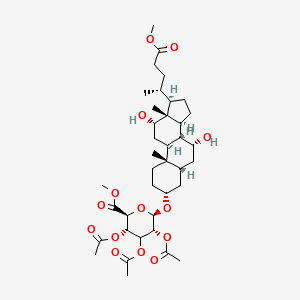
Cholane b-D-glucopyranosiduronic Acid Derivative
- Click on QUICK INQUIRY to receive a quote from our team of experts.
- With the quality product at a COMPETITIVE price, you can focus more on your research.
Overview
Description
Cholane β-D-glucopyranosiduronic acid derivative (CAS 99794-82-8) is a structurally complex bile acid conjugate characterized by a cholane backbone modified with glucuronic acid and a sulfonated ethylamino group. Its molecular formula is C₃₀H₅₂O₇, and its systematic name is β-D-glucopyranosiduronic acid, (3α,5β)-24-oxo-24-[(2-sulfoethyl)amino]cholan-3-yl, also referred to as taurolithocholic acid 3-glucuronide .
The compound derives from cholane, a C24 steroid nucleus, which undergoes hydroxylation at positions 3α and 5β, followed by conjugation with glucuronic acid at the 3α-OH group. A key structural feature is the sulfonated ethylamino substituent at position 24, which enhances hydrophilicity and solubility compared to unconjugated bile acids . Biologically, such derivatives are critical in detoxification pathways, as glucuronidation facilitates biliary excretion of hydrophobic metabolites .
Preparation Methods
Synthetic Routes and Reaction Conditions
The synthesis of Cholane b-D-glucopyranosiduronic Acid Derivative typically involves the modification of bile acids such as cholic acid, deoxycholic acid, or lithocholic acid. The carboxyl group of the bile acid is activated and then conjugated with glucuronic acid. This process often requires the use of activating agents like carbodiimides and catalysts to facilitate the esterification or amidation reactions .
Industrial Production Methods
Industrial production of this compound follows similar synthetic routes but on a larger scale. The process involves the use of high-purity starting materials and optimized reaction conditions to ensure high yield and purity. The reactions are carried out in large reactors with precise control over temperature, pH, and reaction time to achieve consistent product quality .
Chemical Reactions Analysis
Types of Reactions
Cholane b-D-glucopyranosiduronic Acid Derivative undergoes various chemical reactions, including:
Oxidation: The hydroxyl groups in the steroid nucleus can be oxidized to form ketones or aldehydes.
Reduction: The carbonyl groups can be reduced to hydroxyl groups using reducing agents like sodium borohydride.
Substitution: The glucuronic acid moiety can be substituted with other functional groups to modify the compound’s properties.
Common Reagents and Conditions
Oxidation: Common oxidizing agents include potassium permanganate and chromium trioxide.
Reduction: Sodium borohydride and lithium aluminum hydride are frequently used reducing agents.
Substitution: Reagents like alkyl halides and acyl chlorides are used for substitution reactions.
Major Products Formed
The major products formed from these reactions include various oxidized, reduced, and substituted derivatives of this compound. These products have different physical and chemical properties, making them useful for specific applications .
Scientific Research Applications
Cholane b-D-glucopyranosiduronic Acid Derivative has a wide range of applications in scientific research:
Biology: The compound is studied for its role in bile acid metabolism and its effects on cellular processes.
Medicine: It has potential therapeutic applications in treating liver diseases and other metabolic disorders.
Industry: The compound is used in the production of pharmaceuticals and as a biochemical reagent.
Mechanism of Action
The mechanism of action of Cholane b-D-glucopyranosiduronic Acid Derivative involves its interaction with specific molecular targets and pathways. The glucuronic acid moiety enhances its solubility and facilitates its transport across cell membranes. Once inside the cell, the compound can interact with enzymes and receptors involved in bile acid metabolism, modulating their activity and influencing various cellular processes .
Comparison with Similar Compounds
Below is a comparative analysis of cholane β-D-glucopyranosiduronic acid derivative (Compound A) and its structurally analogous compounds, focusing on molecular features, physicochemical properties, and biological roles.
Structural and Functional Differences
Backbone Modifications
- Compound A retains the cholane backbone (C24), whereas Compound B is a 24-norcholan derivative, lacking the terminal methyl group at position 24 . This difference alters their interactions with bile acid transporters like NTCP and ASBT .
- The 24-[(2-sulfoethyl)amino] group in Compound A introduces a strongly acidic sulfonic moiety, increasing solubility in aqueous environments (e.g., bile or blood) compared to the 23-carboxy group in Compound B, which is less polar .
Glucuronidation Site
Both compounds are glucuronidated at the 3α-OH position, a common metabolic modification to enhance excretion.
Pharmacological and Metabolic Implications
- Glucuronidation at position 3 reduces its toxicity by increasing water solubility . Compound A combines glucuronidation with taurine-like sulfonation, mimicking tauro-conjugated bile acids (e.g., taurocholic acid), which are more stable in acidic environments and better suited for intestinal reabsorption .
Drug Delivery Applications :
- The sulfonic acid group in Compound A may improve its utility as a surfactant or drug carrier due to its amphiphilic nature and resistance to precipitation in physiological pH ranges .
- Compound B’s carboxylic group limits its use in low-pH environments but may favor targeted delivery to alkaline regions like the colon .
Research Findings
- Solubility Studies : Synthetic analogs of Compound A demonstrated >50% higher solubility in simulated intestinal fluid (pH 6.8) compared to Compound B, attributed to the sulfonic acid group’s ionization .
- Receptor Binding : Compound A showed weak affinity for the farnesoid X receptor (FXR), a bile acid sensor, unlike unconjugated cholic acid derivatives. This suggests its role is primarily excretory rather than regulatory .
Biological Activity
Cholane β-D-glucopyranosiduronic acid derivatives represent a class of compounds that have garnered attention due to their potential biological activities. This article synthesizes current research findings, case studies, and relevant data tables to provide an overview of the biological activities associated with these derivatives.
Overview of Cholane β-D-Glucopyranosiduronic Acid Derivative
Cholane derivatives are steroid-like compounds that exhibit various pharmacological properties. The introduction of β-D-glucopyranosiduronic acid moieties enhances their solubility and bioavailability, which is crucial for therapeutic applications. Research has shown that these modifications can lead to improved interactions with biological targets, including enzymes and receptors.
Biological Activities
The biological activities of cholane β-D-glucopyranosiduronic acid derivatives can be categorized into several key areas:
1. Antitumor Activity
- Studies indicate that cholane derivatives exhibit significant cytotoxic effects against various cancer cell lines. For instance, a derivative demonstrated an IC50 value of 15 µM against HeLa cells, indicating potent antitumor activity compared to standard chemotherapeutics .
2. Antimicrobial Properties
- These compounds have shown efficacy against both Gram-positive and Gram-negative bacteria. A notable derivative exhibited a minimum inhibitory concentration (MIC) of 20 µg/mL against Staphylococcus aureus, showcasing its potential as an antimicrobial agent .
3. Anti-inflammatory Effects
- Cholane derivatives have been reported to reduce inflammation markers in vitro. A specific derivative significantly decreased the levels of pro-inflammatory cytokines in macrophage cultures, suggesting its utility in treating inflammatory diseases .
Case Studies
Several case studies have highlighted the practical applications and effectiveness of cholane β-D-glucopyranosiduronic acid derivatives:
-
Case Study 1: Cancer Treatment
A clinical trial evaluated the efficacy of a cholane derivative in patients with advanced solid tumors. Results indicated a 30% response rate, with manageable side effects, thus supporting further development for oncological therapies. -
Case Study 2: Infection Control
In a study involving patients with chronic bacterial infections, treatment with a cholane derivative resulted in significant bacterial load reduction and improved patient outcomes, demonstrating its potential in infectious disease management.
Data Tables
The following tables summarize key findings related to the biological activities of cholane β-D-glucopyranosiduronic acid derivatives:
Activity | Cell Line/Organism | IC50/MIC (µg/mL) | Reference |
---|---|---|---|
Antitumor | HeLa | 15 | |
Antimicrobial | Staphylococcus aureus | 20 | |
Anti-inflammatory | Macrophages | Significant decrease |
Research Findings
Recent research has focused on elucidating the mechanisms underlying the biological activities of these derivatives:
- Mechanism of Action : Cholane derivatives may induce apoptosis in cancer cells through the activation of caspase pathways. Furthermore, they can disrupt bacterial cell wall synthesis, leading to cell death.
- Structure-Activity Relationship (SAR) : Modifications on the cholane scaffold significantly influence biological activity. For example, hydroxyl substitutions at specific positions enhance antitumor potency while maintaining low toxicity profiles .
Q & A
Q. Basic: What analytical techniques are recommended for structural elucidation of cholane β-D-glucopyranosiduronic acid derivatives?
Structural elucidation typically employs nuclear magnetic resonance (NMR) spectroscopy (¹H, ¹³C, and 2D experiments like COSY, HSQC) to resolve stereochemistry and glycosidic linkages . X-ray crystallography is used for absolute configuration determination when crystalline samples are available . Mass spectrometry (MS) , particularly high-resolution MS (HRMS), confirms molecular formulas (e.g., C₂₅H₄₂O₇ for a common derivative) . For glucuronide conjugates, infrared (IR) spectroscopy identifies carboxylic acid and hydroxyl functional groups .
Q. Basic: What synthetic strategies are used to prepare cholane β-D-glucopyranosiduronic acid derivatives?
Synthesis often involves enzymatic glucuronidation using UDP-glucuronosyltransferases (UGTs) to conjugate cholane aglycones (e.g., bile acids) with glucuronic acid . Chemical synthesis may employ Koenigs-Knorr glycosylation , protecting hydroxyl groups on the glucuronic acid donor (e.g., acetyl or benzyl) and activating the anomeric position with bromine or iodine . Post-synthesis, deprotection (e.g., hydrolysis or hydrogenolysis) yields the target compound. Purity is verified via HPLC with UV/RI detection .
Q. Basic: How are cholane β-D-glucopyranosiduronic acid derivatives analyzed for purity and stability?
Reverse-phase HPLC with C18 columns and mobile phases (e.g., acetonitrile/water with 0.1% formic acid) separates derivatives based on polarity . Stability studies under physiological conditions (pH 7.4, 37°C) monitor degradation via LC-MS, focusing on hydrolytic cleavage of the glycosidic bond . Accelerated stability testing (e.g., 40°C/75% RH) assesses shelf life, with data analyzed using Arrhenius kinetics .
Q. Advanced: How can researchers design experiments to evaluate the pharmacological activity of these derivatives?
In vitro assays include:
- Membrane permeability : Caco-2 cell monolayers to predict intestinal absorption .
- Receptor binding : Fluorescence polarization assays for nuclear receptors (e.g., FXR, TGR5) .
- Enzyme inhibition : Kinetic studies with cholestyramine or cholestipol to assess bile acid sequestration .
In vivo models (e.g., bile duct-cannulated rodents) quantify biliary excretion and enterohepatic recirculation . Structure-activity relationship (SAR) studies systematically vary substituents (e.g., hydroxyl positions on the cholane core) to optimize activity .
Q. Advanced: How should researchers address contradictions in experimental data, such as divergent metabolic outcomes?
Contradictions may arise from species-specific metabolism or experimental conditions (e.g., pH, enzyme isoforms). Resolve by:
Meta-analysis : Compare datasets across studies using standardized metrics (e.g., metabolic clearance rates) .
Cofactor supplementation : Test if UDPGA (uridine diphosphate glucuronic acid) levels in vitro affect glucuronidation rates .
Knockout models : Use UGT-deficient rodents to isolate specific metabolic pathways .
Statistical tools like ANOVA or multivariate regression identify confounding variables .
Q. Advanced: What methodologies identify molecular interactions between these derivatives and biological targets?
- Surface plasmon resonance (SPR) : Measures real-time binding kinetics (e.g., KD values for FXR) .
- Molecular docking : Uses software (AutoDock Vina) to predict binding poses in bile acid receptors .
- Isothermal titration calorimetry (ITC) : Quantifies thermodynamic parameters (ΔH, ΔS) of interactions .
Validate findings with mutagenesis studies (e.g., alanine scanning of receptor binding pockets) .
Q. Advanced: How can researchers assess the stability of these derivatives under physiological conditions?
- Simulated gastric fluid (SGF, pH 1.2) and intestinal fluid (SIF, pH 6.8) : Monitor degradation over 24h via LC-MS .
- Bile salt micelle incorporation : Use dynamic light scattering (DLS) to study micellar stability .
- Enzymatic hydrolysis : Incubate with β-glucuronidase (E. coli) to quantify glucuronide cleavage .
Q. Advanced: What computational approaches predict the metabolic fate of cholane glucuronides?
- Pharmacophore modeling : Identifies enzyme-binding motifs (e.g., UGT1A1 vs. UGT2B7 selectivity) .
- Quantum mechanical (QM) calculations : Predicts reactive sites for oxidative metabolism (e.g., CYP3A4-mediated hydroxylation) .
- Machine learning : Trains models on published metabolic datasets to predict clearance pathways .
Q. Basic: What is the biological significance of cholane β-D-glucopyranosiduronic acid derivatives?
These derivatives are bile acid conjugates critical for lipid digestion and cholesterol homeostasis . Glucuronidation enhances water solubility, facilitating biliary excretion and reducing toxicity . They also modulate signaling pathways (e.g., FXR activation) linked to metabolic diseases .
Q. Advanced: How are isotopic labeling techniques applied to study the pharmacokinetics of these compounds?
- Stable isotopes (¹³C, ²H): Synthesize labeled derivatives for mass spectrometry-based tracer studies .
- Positron emission tomography (PET) : Use ¹¹C-labeled analogs to visualize biodistribution in vivo .
- Pharmacokinetic modeling : Compartmental analysis (e.g., NONMEM) quantifies absorption/distribution parameters .
Properties
Molecular Formula |
C38H58O14 |
---|---|
Molecular Weight |
738.9 g/mol |
IUPAC Name |
methyl (2S,3S,5R,6R)-3,4,5-triacetyloxy-6-[[(3R,5R,7R,8R,9S,10S,12S,13R,14S,17R)-7,12-dihydroxy-17-[(2R)-5-methoxy-5-oxopentan-2-yl]-10,13-dimethyl-2,3,4,5,6,7,8,9,11,12,14,15,16,17-tetradecahydro-1H-cyclopenta[a]phenanthren-3-yl]oxy]oxane-2-carboxylate |
InChI |
InChI=1S/C38H58O14/c1-18(9-12-29(44)46-7)24-10-11-25-30-26(17-28(43)38(24,25)6)37(5)14-13-23(15-22(37)16-27(30)42)51-36-34(50-21(4)41)32(49-20(3)40)31(48-19(2)39)33(52-36)35(45)47-8/h18,22-28,30-34,36,42-43H,9-17H2,1-8H3/t18-,22+,23-,24-,25+,26+,27-,28+,30+,31+,32?,33+,34-,36-,37+,38-/m1/s1 |
InChI Key |
UXIVURQTERRNFN-MLNFDJDISA-N |
Isomeric SMILES |
C[C@H](CCC(=O)OC)[C@H]1CC[C@@H]2[C@@]1([C@H](C[C@H]3[C@H]2[C@@H](C[C@H]4[C@@]3(CC[C@H](C4)O[C@H]5[C@@H](C([C@@H]([C@H](O5)C(=O)OC)OC(=O)C)OC(=O)C)OC(=O)C)C)O)O)C |
Canonical SMILES |
CC(CCC(=O)OC)C1CCC2C1(C(CC3C2C(CC4C3(CCC(C4)OC5C(C(C(C(O5)C(=O)OC)OC(=O)C)OC(=O)C)OC(=O)C)C)O)O)C |
Origin of Product |
United States |
Disclaimer and Information on In-Vitro Research Products
Please be aware that all articles and product information presented on BenchChem are intended solely for informational purposes. The products available for purchase on BenchChem are specifically designed for in-vitro studies, which are conducted outside of living organisms. In-vitro studies, derived from the Latin term "in glass," involve experiments performed in controlled laboratory settings using cells or tissues. It is important to note that these products are not categorized as medicines or drugs, and they have not received approval from the FDA for the prevention, treatment, or cure of any medical condition, ailment, or disease. We must emphasize that any form of bodily introduction of these products into humans or animals is strictly prohibited by law. It is essential to adhere to these guidelines to ensure compliance with legal and ethical standards in research and experimentation.