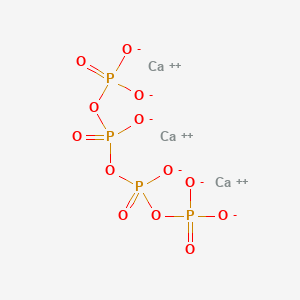
Calcium phosphate, tetra
- Click on QUICK INQUIRY to receive a quote from our team of experts.
- With the quality product at a COMPETITIVE price, you can focus more on your research.
Overview
Description
Tetracalcium phosphate (TTCP), with the chemical formula Ca₄(PO₄)₂O, is a calcium phosphate compound characterized by a Ca/P molar ratio of 2.0, the highest among common calcium phosphates . TTCP is metastable in aqueous environments, hydrolyzing to form HA under physiological conditions, a property exploited in calcium phosphate cements (CPCs) for bone regeneration .
Preparation Methods
Preparation Methods of Tetracalcium Phosphate
Solid-State Reaction Method
This is the classical and most common method for TTCP preparation.
- Process : Stoichiometric mixtures of calcium phosphate salts (e.g., dicalcium phosphate, calcium carbonate) are mixed and calcined at high temperatures between 1300°C and 1500°C for several hours.
- Typical Reaction :
$$
2 \text{Ca}3(\text{PO}4)2 + \text{CaCO}3 \rightarrow \text{Ca}4(\text{PO}4)2\text{O} + \text{CO}2 \uparrow
$$ - Advantages : Established method, scalable.
- Disadvantages : High temperature leads to energy consumption; often results in TTCP mixed with hydroxyapatite and calcium oxide impurities; requires post-synthesis grinding which can introduce contamination and reduce bioactivity.
Example from Patent CN101264871A :
- Natural bone powder mixed with calcium carbonate in a molar Ca/P ratio of 2–2.1.
- Suspension formed, dried, ball-milled in ethanol for 3 hours to increase surface area and reactivity.
- Calcination at 1400–1500°C for several hours.
- Result: High-purity TTCP with reduced impurities after controlled cooling and quenching.
Step | Description | Conditions |
---|---|---|
Mixing | Natural bone powder + CaCO3 | Molar Ca/P 2–2.1, distilled water |
Suspension | Formation of hydroxyapatite-calcium carbonate suspension | Stirring, drying at 80–120°C |
Ball milling | Ethanol medium, 3 hours | Enhances contact and surface area |
Calcination | Sintering furnace | 1400–1500°C, 4–6 hours |
Cooling | Air quenching | Prevents unwanted phase formation |
Calcination of Coprecipitated Nanocrystalline Precursors
Recent research has shown that nanoscale precursors can reduce the required calcination temperature and improve TTCP purity.
- Process : Hydroxyapatite (HA) nanoparticles and calcium carbonate (CaCO3) crystals are coprecipitated and mixed uniformly.
- Calcination : Heated above 1185°C, with optimal purity achieved near 1450°C.
- Cooling : Air quenching favors TTCP formation; furnace cooling leads to HA and CaO mixture.
- Advantages : Lower calcination temperature due to nanoscale precursors; better control of phase purity.
- Disadvantages : Requires precise control of precipitation and calcination parameters.
Key findings from Liao et al. (2014) :
- TTCP begins to form above 1185°C.
- Intermediate phases disappear at 1450°C.
- Cooling method critically affects phase composition.
- Nanoscale precursors enhance reactivity and reduce temperature requirements.
Parameter | Effect on TTCP Formation |
---|---|
Calcination Temperature | >1185°C initiates TTCP; 1450°C yields pure TTCP |
Cooling Method | Air quenching produces TTCP; furnace cooling yields HA + CaO |
Precursor Size | Nanoscale reduces temperature and improves purity |
Low-Temperature Synthesis via Chemical Routes
A novel method uses calcium acetate monohydrate and ammonium dihydrogen phosphate as calcium and phosphate sources, respectively.
- Process :
- Mix Ca(CH3COO)2·H2O and NH4H2PO4 powders.
- Heat gradually: NH4H2PO4 melts (~190°C), calcium acetate decomposes to CaCO3 (~400°C).
- Further heating leads to formation of hydroxyapatite and β-tricalcium phosphate (~700°C).
- TTCP phase forms slightly above 1200°C.
- Advantages :
- Lowest reported synthesis temperature (~1230°C).
- Reduced grinding requirements.
- In situ seeding with hydroxyapatite enhances bioactivity.
- Disadvantages : Complex thermal decomposition steps; requires careful thermal management.
From Cuneyt Tas et al. (2014) :
The multi-stage thermal decomposition and melting process leads to a highly reactive intermediate mixture, facilitating TTCP formation at lower temperatures than traditional solid-state methods.
Temperature Range (°C) | Thermal Event | Resulting Phase |
---|---|---|
~190 | NH4H2PO4 melts | Molten phosphate phase engulfs Ca acetate |
~400 | Ca acetate decomposes | Formation of CaCO3 and amorphous Ca-metaphosphate |
~700 | Crystallization | Hydroxyapatite and β-Ca3(PO4)2 form |
>1200 | TTCP formation | Tetracalcium phosphate phase appears |
Comparative Summary of Preparation Methods
Method | Temperature Range (°C) | Precursors | Cooling | Advantages | Disadvantages |
---|---|---|---|---|---|
Solid-State Reaction | 1300–1500 | Calcium phosphate salts, CaCO3 | Air quenching | Established, scalable | High temp, impurities, grinding needed |
Nanocrystalline Coprecipitation + Calcination | 1185–1450 | Nanoscale HA + CaCO3 | Air quenching preferred | Lower temp, high purity | Requires nanoscale control |
Chemical Route with Ca Acetate & NH4H2PO4 | ~1230 | Ca(CH3COO)2·H2O + NH4H2PO4 | Controlled heating | Lowest temp, less grinding, HA-seeded | Complex thermal steps |
Research Findings and Process Optimization
- Calcination temperature and cooling rate are critical : Air quenching prevents decomposition into undesired phases.
- Particle size of precursors influences reaction kinetics : Nanosized precursors lower activation energy and temperature.
- Ball milling with ethanol enhances reactivity by increasing surface area and homogeneity.
- Thermal analysis (DTA/TG), XRD, FTIR, and Raman spectroscopy are essential tools for monitoring phase evolution and purity.
- Hydroxyapatite seeding during synthesis improves bioactivity of the final TTCP powder.
Chemical Reactions Analysis
Types of Reactions
Tetracalcium phosphate undergoes several types of chemical reactions, including hydrolysis and solid-state reactions. It is stable in water at room temperature for up to four weeks but hydrolyzes to hydroxyapatite and calcium hydroxide at higher temperatures . The hydrolysis reaction is as follows: [ 3 \text{Ca}_4(\text{PO}_4)_2\text{O} + 3 \text{H}_2\text{O} \rightarrow 2 \text{Ca}_5(\text{PO}_4)_3(\text{OH}) + 2 \text{Ca}(\text{OH})_2 ]
Common Reagents and Conditions
Common reagents used in reactions with tetracalcium phosphate include calcium hydrogen phosphate and calcium carbonate. The reactions typically require high temperatures (above 1300°C) and rapid quenching to maintain the desired phase .
Major Products Formed
The major products formed from reactions involving tetracalcium phosphate include hydroxyapatite (Ca₅(PO₄)₃(OH)) and calcium hydroxide (Ca(OH)₂) . These products are significant in medical applications, particularly in bone repair and regeneration.
Scientific Research Applications
Tetracalcium phosphate (TTCP) is used in a variety of applications, including medical and non-medical fields . TTCP has unique properties that make it useful in bone repair, remineralization, and drug delivery .
Scientific Research Applications
Bone Repair: TTCP is a component in hydroxyapatite calcium phosphate cements used for bone defect repair . When mixed with dicalcium phosphate anhydrous, it converts to hydroxyapatite several hours after mixing .
Dentin Permeability Reduction: Dicalcium and tetracalcium phosphate-based desensitizers can reduce dentin permeability by occluding tubules . A study showed that a calcium phosphate-based desensitizer consistently reduced dentin permeability by 50% after 24 hours when applied three times .
Biomimetic Oral Care: Calcium phosphates, including TTCP, are used in biomimetic oral care for remineralizing early caries lesions and repairing small enamel defects . Beta-tricalcium phosphate (β-TCP) increases calcium concentration in saliva, aiding remineralization .
Drug Delivery: Calcium phosphate nanoparticles are used as non-viral vectors for gene and drug delivery in biological systems . The mechanism involves cellular uptake, endosome fusion with lysosomes, nanoparticle degradation, and release of cargo into the cytosol .
Studies
An in vivo study showed that chewing gums with 2.5% β-TCP increased calcium in saliva and buffered acidic attacks . Another study found that CPP-ACP (casein phosphopeptide-amorphous calcium phosphate) reduced the percentage of Streptococcus mutans in children .
Table: Applications of Calcium Phosphates
Mechanism of Action
Tetracalcium phosphate exerts its effects primarily through the release of calcium and phosphate ions. These ions play a crucial role in bone homeostasis and remineralization . In medical applications, the compound provides a source of calcium and phosphate ions that support bone regeneration and repair . The molecular targets and pathways involved include the activation of osteoblasts and osteoclasts, which are essential for bone formation and resorption .
Comparison with Similar Compounds
Comparison with Similar Calcium Phosphate Compounds
Structural and Compositional Differences
Calcium phosphates are categorized by their Ca/P ratios , solubility, and crystallinity. Below is a comparative analysis of TTCP with key analogs:
Key Observations :
- Ca/P Ratio : TTCP has the highest Ca/P ratio (2.0), making it distinct from HA (1.67) and β-TCP (1.5). This ratio influences solubility and reactivity .
- Solubility : TTCP is more soluble than HA (Ksp ~0.0007 vs. ~0.0003) but less soluble than OCP (Ksp ~0.008), which governs its hydrolysis kinetics in biological environments .
- Synthesis: Unlike HA or OCP, TTCP cannot be precipitated from aqueous solutions and requires non-aqueous methods .
Solubility and Reactivity
TTCP’s higher solubility compared to HA enables its use in self-setting cements. When combined with dicalcium phosphate anhydrous (DCPA), TTCP reacts with water to form nanocrystalline HA, providing mechanical strength and biocompatibility . In contrast, HA’s low solubility limits its direct use in cements but enhances durability in coatings .
β-TCP, with intermediate solubility (Ksp ~0.0005), degrades faster than TTCP and HA, making it suitable for temporary bone grafts . OCP, a precursor to HA, has plate-like crystals that promote osteoblast adhesion but lacks TTCP’s cement-forming capability .
Industrial and Commercial Relevance
TTCP is a niche product in the calcium phosphate market, with applications in dental and orthopedic cements . In contrast, HA and β-TCP dominate the broader market due to their versatility in coatings, drug delivery, and food additives .
Biological Activity
Tetracalcium phosphate (TTCP), with the chemical formula Ca4(PO4)2O, is a calcium phosphate compound that plays a significant role in biological systems, particularly in bone regeneration and dental applications. Its unique properties make it a subject of extensive research in biomedical fields, including tissue engineering and drug delivery systems.
TTCP exhibits several biological activities that contribute to its efficacy in medical applications:
- Osteoconductivity : TTCP supports the attachment and proliferation of osteoblasts, which are essential for bone formation. This property is attributed to its ability to release calcium ions, promoting osteogenic differentiation and enhancing alkaline phosphatase activity, a marker for osteoblast function .
- Biocompatibility : Studies have demonstrated that TTCP is biocompatible and does not elicit significant inflammatory responses when implanted in biological tissues. This characteristic is crucial for materials used in bone grafts and implants .
- Hydrolysis : Upon exposure to physiological conditions, TTCP undergoes hydrolysis, leading to the formation of hydroxyapatite (HA), which is the primary mineral component of bone. This conversion enhances its integration with surrounding bone tissue .
Applications in Medicine
TTCP is utilized in various biomedical applications due to its favorable biological properties:
- Bone Cements : TTCP is commonly used in self-curing bone cements, which are employed in orthopedic surgeries and dental procedures. These cements provide mechanical support while facilitating bone healing .
- Bone Grafting : As a component of bioactive ceramics, TTCP is incorporated into composite materials for bone grafting, enhancing the material's mechanical strength and biological performance .
- Drug Delivery Systems : Recent research highlights the potential of TTCP as a nanocarrier for drug delivery. Its ability to encapsulate therapeutic agents and release them in a controlled manner makes it suitable for targeted therapies .
Case Studies
-
Bone Regeneration in Animal Models :
A study involving rabbit models demonstrated that TTCP-based composites significantly improved bone regeneration compared to control groups. Histological analysis revealed enhanced osteoconductivity and new bone formation at 12 weeks post-implantation . -
Dental Applications :
In dental procedures, TTCP has been shown to promote healing in periodontal defects. Clinical trials indicated that patients treated with TTCP-based grafts exhibited improved clinical outcomes and radiographic evidence of bone fill compared to those receiving conventional treatments .
Summary of Key Studies
Q & A
Basic Research Questions
Q. What are the primary challenges in synthesizing pure tetra-calcium phosphate (Ca₄(PO₄)₂O) crystals, and how can contamination be minimized?
Synthesizing pure tetra-calcium phosphate requires strict control of reaction conditions (e.g., pH, temperature, and ion concentrations) to avoid contamination by metallic oxides or other calcium phosphate phases (e.g., hydroxyapatite). Evidence suggests that impurities often arise from incomplete purification or interactions with atmospheric CO₂, leading to carbonate apatite formation. To minimize contamination, use inert atmospheres (e.g., nitrogen), high-purity precursors, and iterative recrystallization steps. Analytical techniques like X-ray diffraction (XRD) and energy-dispersive X-ray spectroscopy (EDS) should validate phase purity .
Q. What analytical methods are recommended for characterizing the crystallinity and phase composition of tetra-calcium phosphate?
A combination of XRD (to confirm crystal structure), Fourier-transform infrared spectroscopy (FT-IR) (to identify phosphate and hydroxyl groups), and thermogravimetric analysis (TGA) (to assess thermal stability) is essential. Scanning electron microscopy (SEM) or transmission electron microscopy (TEM) can resolve particle morphology and surface defects. Solubility assays in simulated physiological fluids (e.g., Tris buffer) provide insights into stability under biological conditions .
Q. How does the solubility of tetra-calcium phosphate compare to other calcium phosphates, and why is this critical for biomaterial applications?
Tetra-calcium phosphate is less soluble than hydroxyapatite (HAp) but more soluble than tricalcium phosphate (TCP) under physiological pH. This intermediate solubility makes it suitable for bone regeneration, as it balances resorption and osteoconductivity. Solubility can be quantified via ion-selective electrode measurements or inductively coupled plasma mass spectrometry (ICP-MS) to track Ca²⁺ and PO₄³⁻ release kinetics .
Advanced Research Questions
Q. How can researchers address contradictions in solubility data for tetra-calcium phosphate across different studies?
Discrepancies often arise from variations in experimental conditions (e.g., buffer composition, ionic strength) or contamination. Standardize protocols by using controlled environments (e.g., ISO-certified labs) and reference materials (e.g., NIST-certified HAp). Cross-validate results with multiple techniques, such as XRD for phase identification and ICP-MS for ion quantification. Meta-analyses of published data can identify systematic biases .
Q. What experimental strategies mitigate false positives in protein interaction studies involving tetra-calcium phosphate precipitation?
Calcium phosphate precipitates can nonspecifically bind proteins, leading to false positives. To avoid this:
- Use buffers with neutral pH (e.g., Tris-HCl) instead of alkaline conditions.
- Include negative controls with calcium chelators (e.g., EDTA).
- Employ orthogonal validation methods like Western blotting or mass spectrometry .
Q. How do organic molecules (e.g., collagen, citrate) influence the co-precipitation of tetra-calcium phosphate in biomimetic systems?
Organic additives act as templating agents, directing crystal growth and stabilizing metastable phases. For example, citrate ions inhibit HAp formation by binding Ca²⁺, favoring tetra-calcium phosphate precipitation. Experimental designs should incorporate in situ FT-IR or Raman spectroscopy to monitor real-time interactions between organic molecules and growing crystals .
Q. What methodologies are effective for evaluating the biocompatibility of tetra-calcium phosphate scaffolds in vitro?
- Cell proliferation assays (e.g., MTT or Alamar Blue) using osteoblast-like cells (e.g., MC3T3-E1).
- Cell morphology analysis via SEM or confocal microscopy to assess adhesion and spreading.
- Osteogenic differentiation markers (e.g., alkaline phosphatase activity, osteocalcin ELISA).
Ensure scaffolds are sterilized via autoclaving or gamma irradiation to prevent endotoxin contamination .
Q. How can collaborative frameworks improve the reproducibility of tetra-calcium phosphate research?
Adopt open-science practices:
- Share raw data and protocols via repositories like Zenodo or Figshare.
- Use standardized characterization checklists (e.g., MIABiotech for biomaterials).
- Engage in cross-lab validation studies to identify protocol-specific artifacts .
Q. Methodological Recommendations
- Synthesis : Use sol-gel methods with Ca(NO₃)₂·4H₂O and (NH₄)₂HPO₄ precursors for high purity .
- Data Interpretation : Apply Rietveld refinement to XRD data to quantify phase mixtures .
- Ethical Compliance : Follow institutional guidelines for human-derived samples (e.g., informed consent for in vitro studies using dental tissues) .
Properties
Molecular Formula |
Ca3O13P4 |
---|---|
Molecular Weight |
452.12 g/mol |
IUPAC Name |
tricalcium;[oxido-[oxido(phosphonatooxy)phosphoryl]oxyphosphoryl] phosphate |
InChI |
InChI=1S/3Ca.H6O13P4/c;;;1-14(2,3)11-16(7,8)13-17(9,10)12-15(4,5)6/h;;;(H,7,8)(H,9,10)(H2,1,2,3)(H2,4,5,6)/q3*+2;/p-6 |
InChI Key |
YSJNWPJHMDWGAA-UHFFFAOYSA-H |
Canonical SMILES |
[O-]P(=O)([O-])OP(=O)([O-])OP(=O)([O-])OP(=O)([O-])[O-].[Ca+2].[Ca+2].[Ca+2] |
Origin of Product |
United States |
Disclaimer and Information on In-Vitro Research Products
Please be aware that all articles and product information presented on BenchChem are intended solely for informational purposes. The products available for purchase on BenchChem are specifically designed for in-vitro studies, which are conducted outside of living organisms. In-vitro studies, derived from the Latin term "in glass," involve experiments performed in controlled laboratory settings using cells or tissues. It is important to note that these products are not categorized as medicines or drugs, and they have not received approval from the FDA for the prevention, treatment, or cure of any medical condition, ailment, or disease. We must emphasize that any form of bodily introduction of these products into humans or animals is strictly prohibited by law. It is essential to adhere to these guidelines to ensure compliance with legal and ethical standards in research and experimentation.