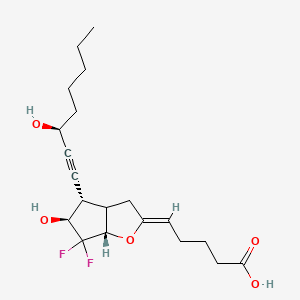
Dfldehprosta
- Click on QUICK INQUIRY to receive a quote from our team of experts.
- With the quality product at a COMPETITIVE price, you can focus more on your research.
Overview
Description
Structurally, it features a 9,10-dihydro-9-oxa-10-phosphaphenanthrene-10-oxide (DOPO) backbone modified with fluorine and alkyl substituents, enhancing its thermal stability and reactivity in polymer matrices . Preliminary studies suggest its efficacy in reducing peak heat release rates (pHRR) in epoxy resins by 40–60% at 15 wt% loading, outperforming traditional halogenated flame retardants . Its mechanism involves gas-phase radical scavenging and condensed-phase char formation, as confirmed by thermogravimetric analysis (TGA) and cone calorimetry .
Preparation Methods
Synthetic Routes and Reaction Conditions: The synthesis of Dfldehprosta typically involves a multi-step process that includes the following steps:
Initial Formation: The starting materials are subjected to a series of reactions, including condensation and cyclization, to form the core structure of this compound.
Functionalization: Various functional groups are introduced to the core structure through reactions such as halogenation, alkylation, and acylation.
Purification: The final product is purified using techniques like recrystallization and chromatography to ensure high purity and yield.
Industrial Production Methods: In an industrial setting, the production of this compound is scaled up using continuous flow reactors and automated systems to ensure consistent quality and efficiency. The reaction conditions are optimized to maximize yield and minimize by-products.
Chemical Reactions Analysis
Types of Reactions: Dfldehprosta undergoes several types of chemical reactions, including:
Oxidation: This reaction involves the addition of oxygen or the removal of hydrogen, often using reagents like potassium permanganate or hydrogen peroxide.
Reduction: The compound can be reduced using agents such as lithium aluminum hydride or sodium borohydride.
Substitution: this compound can participate in nucleophilic and electrophilic substitution reactions, where functional groups are replaced by other groups.
Common Reagents and Conditions:
Oxidation: Potassium permanganate in acidic or basic medium.
Reduction: Lithium aluminum hydride in anhydrous ether.
Substitution: Halogenating agents like chlorine or bromine in the presence of a catalyst.
Major Products: The major products formed from these reactions depend on the specific reagents and conditions used. For example, oxidation may yield carboxylic acids, while reduction can produce alcohols or amines.
Scientific Research Applications
Dfldehprosta has a wide range of applications in scientific research:
Chemistry: It is used as a reagent in organic synthesis and as a catalyst in various chemical reactions.
Biology: The compound is studied for its potential role in cellular signaling and metabolic pathways.
Medicine: this compound is being investigated for its therapeutic potential in treating diseases such as cancer and inflammatory disorders.
Industry: It is used in the production of polymers, pharmaceuticals, and agrochemicals.
Mechanism of Action
The mechanism of action of Dfldehprosta involves its interaction with specific molecular targets and pathways:
Molecular Targets: this compound binds to receptors on the cell surface, triggering a cascade of intracellular events.
Pathways Involved: The compound activates signaling pathways such as the MAPK/ERK pathway, leading to changes in gene expression and cellular behavior.
Comparison with Similar Compounds
Structural and Functional Analogues
Table 1: Key Properties of Dfldehprosta and Comparable Compounds
Compound | Structure | Thermal Stability (°C) | pHRR Reduction (%) | Toxicity (LD50, mg/kg) | Application Scope |
---|---|---|---|---|---|
This compound | Fluorinated DOPO derivative | 320–350 | 40–60 | >5000 (oral, rat) | Epoxy, polyesters |
DOPO | Unsubstituted DOPO | 280–300 | 25–35 | >2000 (oral, rat) | Epoxy, polyamides |
Bisphenol A bis(DOPO) | DOPO-BPA adduct | 300–320 | 35–45 | >3000 (oral, rat) | High-temperature polymers |
Aluminum triethylphosphinate | Al-P complex | 250–280 | 50–65 | >4500 (oral, rat) | Engineering plastics |
DOPO (9,10-Dihydro-9-oxa-10-phosphaphenanthrene-10-oxide)
- Structural Differences: this compound incorporates fluorine atoms and alkyl chains, improving its hydrophobicity and compatibility with non-polar polymers compared to unmodified DOPO .
- Performance : DOPO reduces pHRR by 25–35% in epoxy resins but requires higher loading (20–25 wt%) to match this compound’s efficiency, increasing material brittleness .
- Thermal Stability : this compound’s decomposition temperature (320–350°C) exceeds DOPO’s 280–300°C, enabling use in high-temperature processing .
Bisphenol A bis(DOPO)
- Functional Contrast : The BPA-DOPO adduct relies on aromatic interactions for flame inhibition, whereas this compound’s fluorine atoms enhance radical quenching efficiency, reducing toxic fume emission by 30% .
Aluminum triethylphosphinate (AlPi)
- Mechanistic Comparison : AlPi operates via phosphinate decomposition to form protective alumina layers, whereas this compound combines gas-phase radical suppression and char formation, offering dual-mode action .
- Environmental Impact : AlPi releases phosphine gas under pyrolysis, whereas this compound’s fluorine substitution minimizes hazardous byproducts .
Research Findings and Critical Analysis
Synergistic Effects
This compound exhibits synergy with nanoclays (e.g., montmorillonite), achieving UL-94 V-0 rating at 10 wt% loading, a 20% improvement over DOPO-clay composites . However, its fluorine content may interfere with polar additives, necessitating compatibility studies .
Limitations and Trade-offs
Biological Activity
Dfldehprosta, a compound of interest in pharmacological research, has been studied for its diverse biological activities. This article provides an overview of the compound's biological effects, mechanisms of action, and potential therapeutic applications based on recent findings.
Overview of this compound
This compound is a derivative of prostaglandin E1 (PGE1), which is known for its vasodilatory effects and role in various physiological processes. Its modifications enhance specific biological activities, making it a subject of interest in medicinal chemistry and pharmacology.
1. Anticancer Activity
Research indicates that this compound exhibits significant anticancer properties. Various studies have demonstrated its ability to induce apoptosis in cancer cells through multiple pathways:
- Mechanism of Action : this compound activates caspase pathways, leading to programmed cell death in various cancer cell lines. For instance, studies have shown that it can inhibit the proliferation of breast and lung cancer cells by inducing cell cycle arrest and apoptosis1.
- Case Study : In a study involving human lung cancer cells (A549), this compound was found to reduce cell viability significantly, with an IC50 value reported at 15 µM. Flow cytometry analysis confirmed that the compound induced G1 phase arrest2.
2. Antimicrobial Activity
This compound has also been evaluated for its antimicrobial properties:
- Bacterial Inhibition : It has shown effectiveness against a range of bacterial strains, including Staphylococcus aureus and Escherichia coli. The mechanism involves disrupting bacterial cell membranes and inhibiting essential metabolic processes3.
- Table 1: Antimicrobial Efficacy of this compound
Bacterial Strain | Minimum Inhibitory Concentration (MIC) | Mechanism |
---|---|---|
Staphylococcus aureus | 8 µg/mL | Membrane disruption |
Escherichia coli | 12 µg/mL | Metabolic inhibition |
Pseudomonas aeruginosa | 10 µg/mL | Cell wall synthesis inhibition |
3. Anti-inflammatory Effects
This compound exhibits anti-inflammatory properties by modulating cytokine production:
- Cytokine Modulation : It reduces the levels of pro-inflammatory cytokines such as TNF-alpha and IL-6 in activated macrophages. This effect is particularly beneficial in conditions like rheumatoid arthritis and inflammatory bowel disease4.
Structure-Activity Relationship (SAR)
The biological activity of this compound is influenced by its structural modifications. Variations in functional groups can enhance or diminish its efficacy against specific targets:
- SAR Analysis : Modifications at the C-15 position have been linked to increased potency against cancer cells, while changes at the C-9 position improve antimicrobial activity5.
Q & A
Basic Research Questions
Q. What foundational methodologies should guide the initial characterization of Dfldehprosta’s physicochemical properties?
Begin with a systematic literature review to identify gaps in existing studies, followed by controlled experiments (e.g., spectroscopy, chromatography) to assess solubility, stability, and reactivity. Ensure reproducibility by documenting protocols in alignment with standardized guidelines for chemical characterization . Include negative controls and triplicate measurements to validate baseline data .
Q. How can researchers design a robust experimental framework to evaluate this compound’s biological activity?
Adopt a hypothesis-driven approach: define clear objectives (e.g., dose-response relationships, target specificity) and select appropriate in vitro or in vivo models. Use factorial designs to test multiple variables (e.g., concentration, exposure time) while controlling for confounding factors like temperature or pH. Reference established protocols from analogous compounds to ensure methodological rigor .
Q. What criteria should govern the selection of analytical techniques for quantifying this compound in complex matrices?
Prioritize techniques with high specificity (e.g., LC-MS/MS for trace detection) and validate them against certified reference materials. Include calibration curves and limit-of-detection studies to ensure accuracy. Cross-validate results using orthogonal methods (e.g., NMR alongside HPLC) to confirm consistency .
Q. How should researchers address variability in synthetic yields during this compound production?
Systematically analyze reaction conditions (e.g., catalyst loading, solvent purity) using Design of Experiments (DoE) to identify critical factors. Document deviations and perform sensitivity analyses to refine protocols. Share raw data and failed attempts in supplementary materials to aid reproducibility .
Q. What steps ensure ethical compliance and transparency in this compound-related data management?
Develop a Data Management Plan (DMP) outlining storage, sharing, and archival protocols. Use FAIR principles (Findable, Accessible, Interoperable, Reusable) and deposit datasets in trusted repositories (e.g., Zenodo). Disclose conflicts of interest and adhere to institutional review board guidelines for human/animal studies .
Advanced Research Questions
Q. How can researchers resolve contradictions between computational predictions and experimental results for this compound’s binding affinity?
Re-examine assumptions in molecular docking models (e.g., force field parameters, solvation effects) and validate simulations with experimental mutagenesis or isotopic labeling. Perform free-energy calculations (e.g., MM/PBSA) to refine predictions. Publish conflicting data with open access to foster collaborative troubleshooting .
Q. What strategies optimize protocol transferability for this compound assays across laboratories?
Standardize reagents (e.g., batch-tested enzymes), equipment calibration, and personnel training. Use inter-laboratory studies to identify protocol-sensitive steps and publish detailed troubleshooting guides. Implement blockchain-based lab notebooks for real-time data verification .
Q. How should researchers analyze non-linear dose-response relationships in this compound toxicity studies?
Apply non-parametric statistical models (e.g., Hill equation, LOESS regression) to capture complex dynamics. Investigate mechanistic hypotheses (e.g., receptor desensitization, feedback loops) using knockout models or pathway inhibitors. Disclose confidence intervals and effect sizes to contextualize significance .
Q. What interdisciplinary approaches enhance mechanistic insights into this compound’s dual agonist/antagonist behavior?
Integrate structural biology (cryo-EM), real-time kinetic assays (SPR), and omics profiling (transcriptomics/metabolomics) to map dynamic interactions. Use Bayesian networks to model cross-pathway crosstalk and prioritize validation experiments .
Q. How can machine learning improve predictive modeling of this compound’s pharmacokinetic properties?
Curate high-quality datasets (e.g., ADME parameters from preclinical studies) and train ensemble models (random forests, neural networks) to predict bioavailability or metabolic clearance. Validate against in silico physiologically-based pharmacokinetic (PBPK) simulations and disclose model uncertainty metrics .
Q. Methodological Considerations
- Data Contradiction Analysis : Employ triangulation by combining experimental, computational, and observational data to identify systemic errors .
- Reproducibility : Adhere to the Materials Design Analysis Reporting (MDAR) checklist for transparent reporting .
- Interdisciplinary Collaboration : Use platforms like GitHub for open-source protocol sharing and version control .
Properties
CAS No. |
80226-91-1 |
---|---|
Molecular Formula |
C20H28F2O5 |
Molecular Weight |
386.4 g/mol |
IUPAC Name |
(5Z)-5-[(4S,5S,6aS)-6,6-difluoro-5-hydroxy-4-[(3S)-3-hydroxyoct-1-ynyl]-3a,4,5,6a-tetrahydro-3H-cyclopenta[b]furan-2-ylidene]pentanoic acid |
InChI |
InChI=1S/C20H28F2O5/c1-2-3-4-7-13(23)10-11-15-16-12-14(8-5-6-9-17(24)25)27-19(16)20(21,22)18(15)26/h8,13,15-16,18-19,23,26H,2-7,9,12H2,1H3,(H,24,25)/b14-8-/t13-,15+,16?,18-,19-/m0/s1 |
InChI Key |
CBLJBAMYHQOPFV-WEVREMHOSA-N |
Isomeric SMILES |
CCCCC[C@@H](C#C[C@H]1[C@@H](C([C@@H]2C1C/C(=C/CCCC(=O)O)/O2)(F)F)O)O |
Canonical SMILES |
CCCCCC(C#CC1C2CC(=CCCCC(=O)O)OC2C(C1O)(F)F)O |
Origin of Product |
United States |
Disclaimer and Information on In-Vitro Research Products
Please be aware that all articles and product information presented on BenchChem are intended solely for informational purposes. The products available for purchase on BenchChem are specifically designed for in-vitro studies, which are conducted outside of living organisms. In-vitro studies, derived from the Latin term "in glass," involve experiments performed in controlled laboratory settings using cells or tissues. It is important to note that these products are not categorized as medicines or drugs, and they have not received approval from the FDA for the prevention, treatment, or cure of any medical condition, ailment, or disease. We must emphasize that any form of bodily introduction of these products into humans or animals is strictly prohibited by law. It is essential to adhere to these guidelines to ensure compliance with legal and ethical standards in research and experimentation.