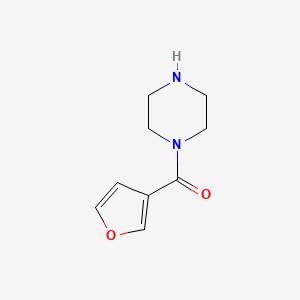
1-(3-Furoyl)piperazine
Overview
Description
1-(3-Furoyl)piperazine is a piperazine derivative featuring a furoyl group (a furan ring substituted with a carbonyl moiety) attached to the piperazine nitrogen. Piperazine derivatives are known for their versatility in drug design due to their ability to interact with neurotransmitter receptors, enzymes, and cellular targets .
Preparation Methods
General Synthetic Approach
The primary synthetic route to 1-(3-furoyl)piperazine involves the reaction of piperazine with 3-furoyl chloride or related activated furoyl derivatives. This acylation reaction typically occurs under controlled conditions to favor monoacylation at one nitrogen atom of piperazine, avoiding diacylation side products.
Reaction Scheme
$$
\text{Piperazine} + \text{3-Furoyl chloride} \xrightarrow[\text{acid acceptor}]{\text{solvent, temp}} 1-(3-\text{Furoyl})\text{piperazine}
$$
The reaction is usually performed in the presence of an acid acceptor (base) such as triethylamine or sodium carbonate to neutralize the hydrochloric acid formed.
Detailed Preparation Method from Literature
Acylation Using 3-Furoyl Chloride
- Reagents : Piperazine (or piperazine hexahydrate), 3-furoyl chloride, acid acceptor (e.g., triethylamine).
- Solvent : Anhydrous solvents such as chloroform, dichloromethane, or glacial acetic acid.
- Conditions : The 3-furoyl chloride is added dropwise to a cooled solution of piperazine and acid acceptor under stirring to maintain temperature around 0–25 °C.
- pH Control : Maintaining a pH around 6.5 to 7 during the reaction is critical to minimize formation of 1,4-di(3-furoyl)piperazine byproduct.
- Workup : After completion, the reaction mixture is diluted with water, and the product is extracted with an inert organic solvent such as chloroform or trichloroethylene.
- Purification : The organic extract is dried and the product is precipitated by introducing dry hydrogen chloride gas to form the hydrochloride salt of this compound, which is then filtered and dried.
Yield and Physical Data
- Yields typically range from 65% to 75% based on the furoyl chloride used.
- The hydrochloride salt melts around 210–215 °C.
- The product is a white crystalline solid.
This method is adapted from a closely related process for 1-(2-furoyl)piperazine and is applicable to the 3-furoyl isomer with minor modifications in reaction parameters.
Alternative Synthetic Routes
Use of 3-Furoic Acid Anhydride or Acid Derivatives
Instead of 3-furoyl chloride, 3-furoic acid anhydride or activated esters can be used to acylate piperazine. This method may require longer reaction times or higher temperatures but can avoid the use of corrosive acid chlorides.
Mannich-Type Reactions Involving Piperazine
Some studies report the synthesis of piperazine derivatives bearing furoyl groups via Mannich base formation involving formaldehyde, piperazine, and furoyl-containing amines or hydrazides. These methods are more complex and typically used for elaborated derivatives rather than simple this compound.
Reaction Parameters and Optimization
Parameter | Typical Conditions | Notes |
---|---|---|
Piperazine form | Anhydrous or hexahydrate | Hexahydrate may require adjustment in solvent |
Solvent | Chloroform, dichloromethane, acetic acid | Choice affects solubility and reaction rate |
Temperature | 0–25 °C | Low temperature controls selectivity |
Acid acceptor | Triethylamine, sodium carbonate | Neutralizes HCl, prevents diacylation |
pH during reaction | 6.5–7.0 | Critical to minimize diacylated byproducts |
Reaction time | 1–3 hours | Monitored by TLC or HPLC |
Workup | Extraction with inert solvent, drying | Precipitation as hydrochloride salt |
Purification and Characterization
- The crude product is purified by extraction and precipitation as hydrochloride salt.
- Characterization includes melting point determination, NMR spectroscopy, and elemental analysis.
- 1H NMR typically shows signals corresponding to the piperazine ring protons and the furoyl aromatic protons.
- The hydrochloride salt form improves stability and handling.
Summary Table of Preparation Methods
Method | Reagents | Conditions | Yield (%) | Notes |
---|---|---|---|---|
Acylation with 3-furoyl chloride | Piperazine, 3-furoyl chloride, acid acceptor | 0–25 °C, inert solvent, pH 6.5–7 | 65–75 | Most common, high purity product |
Acylation with 3-furoic anhydride | Piperazine, 3-furoic anhydride | Elevated temperature, longer time | 50–65 | Alternative to acid chloride |
Mannich-type synthesis | Piperazine, formaldehyde, furoyl amine derivatives | Room temp, ethanol solvent | Variable | Used for complex derivatives, not simple this compound |
Research Findings and Practical Considerations
- Maintaining controlled pH during acylation is essential to avoid diacylated impurities, which complicate purification.
- Use of dry solvents and inert atmosphere improves yield and product quality.
- The hydrochloride salt form is preferred for isolation due to better crystallinity and stability.
- Analytical methods such as TLC, NMR, and melting point are routinely used to confirm product identity and purity.
- The described methods are scalable and suitable for industrial pharmaceutical intermediate production.
Chemical Reactions Analysis
Types of Reactions
1-(3-Furoyl)piperazine undergoes various chemical reactions, including:
Oxidation: The furoyl group can be oxidized to form carboxylic acids or other oxidized derivatives.
Reduction: The compound can be reduced to form corresponding alcohols or amines.
Substitution: The piperazine ring can undergo nucleophilic substitution reactions, leading to the formation of various substituted derivatives.
Common Reagents and Conditions
Oxidation: Common oxidizing agents include potassium permanganate and chromium trioxide.
Reduction: Reducing agents such as lithium aluminum hydride and sodium borohydride are used.
Substitution: Nucleophiles like alkyl halides and acyl chlorides are employed under basic or acidic conditions.
Major Products Formed
The major products formed from these reactions depend on the specific reagents and conditions used. For example, oxidation of the furoyl group may yield furan-2-carboxylic acid, while reduction can produce 3-furanmethanol.
Scientific Research Applications
1-(3-Furoyl)piperazine has a wide range of scientific research applications:
Chemistry: It is used as a building block in the synthesis of more complex molecules, including pharmaceuticals and agrochemicals.
Biology: The compound is studied for its potential biological activities, such as enzyme inhibition and antimicrobial properties.
Medicine: Derivatives of this compound are investigated for their therapeutic potential in treating various diseases, including neurological disorders and infections.
Industry: The compound is utilized in the development of advanced materials, such as polymers and coatings, due to its unique chemical properties.
Mechanism of Action
The mechanism of action of 1-(3-Furoyl)piperazine depends on its specific application. In medicinal chemistry, it may act by binding to specific molecular targets, such as enzymes or receptors, thereby modulating their activity. For example, it can inhibit the activity of certain enzymes by forming stable complexes with their active sites, leading to therapeutic effects.
Comparison with Similar Compounds
Comparison with Structurally Similar Compounds
Structural Analogs and Pharmacological Activities
Key Observations:
- Aromatic Substituent Effects: Electron-withdrawing groups (e.g., -CF₃ in TFMPP) enhance 5-HT1B selectivity, while electron-donating groups (e.g., -OCH₃) favor dopamine receptor interactions .
Receptor Selectivity :
Physicochemical Properties
Table 2: Physicochemical Comparison of Selected Piperazines
Key Observations:
- Furan vs. However, the furan ring may reduce metabolic stability due to susceptibility to enzymatic oxidation .
Spectroscopic Signatures :
- Methoxy and chloro substituents in phenylpiperazines produce distinct FT-IR and NMR profiles, aiding in structural identification .
Biological Activity
1-(3-Furoyl)piperazine is a heterocyclic organic compound that combines a piperazine ring with a furoyl group, making it of significant interest in medicinal chemistry and materials science. The unique structural features of this compound contribute to its diverse biological activities, including antimicrobial, anticancer, and enzyme inhibitory properties. This article reviews the biological activity of this compound, highlighting its mechanisms of action, relevant case studies, and research findings.
Chemical Structure and Properties
This compound has the chemical formula C10H12N2O2 and a molecular weight of approximately 192.22 g/mol. The compound's structure can be represented as follows:
The presence of both the furoyl and piperazine moieties is crucial for its biological activity, influencing its interaction with various biological targets.
The biological activity of this compound can be attributed to its ability to interact with specific molecular targets. It may act through:
- Enzyme Inhibition : By binding to the active sites of enzymes, it can modulate their activity, which is critical in therapeutic applications.
- Antimicrobial Activity : The compound has shown potential in inhibiting the growth of various bacterial strains, particularly Gram-positive bacteria.
- Cytotoxic Effects : Research indicates that derivatives of this compound exhibit cytotoxicity against cancer cell lines, suggesting potential anticancer properties .
Antimicrobial Properties
This compound has been studied for its antimicrobial effects against various pathogens. Notably:
- Gram-positive bacteria : It demonstrated significant inhibitory effects on strains such as Staphylococcus aureus and Micrococcus luteus.
- Gram-negative bacteria : While less effective than against Gram-positive strains, some derivatives showed activity against Salmonella typhimurium and Bordetella bronchiseptica.
The Minimum Inhibitory Concentration (MIC) values for these activities vary but indicate promising potential as an antimicrobial agent .
Anticancer Activity
Research has highlighted the potential of this compound as an anticancer agent. In vitro studies have shown that it can induce apoptosis in cancer cell lines through various mechanisms:
- Cell Cycle Arrest : The compound has been observed to cause cell cycle arrest at the G2/M phase.
- Induction of Apoptosis : It activates apoptotic pathways leading to increased caspase activity in cancer cells.
Study 1: Antimicrobial Efficacy
A study evaluated the antimicrobial efficacy of several furoyl-piperazine derivatives, including this compound. The results indicated that this compound exhibited a broad spectrum of antimicrobial activity, particularly against Gram-positive bacteria. The study utilized disc diffusion methods to assess inhibition zones, confirming its potential as a lead compound for developing new antibiotics .
Study 2: Cytotoxicity Against Cancer Cells
In another investigation, researchers assessed the cytotoxic effects of this compound on human cancer cell lines. The compound was found to significantly reduce cell viability in a dose-dependent manner, with IC50 values indicating strong potential for further development as an anticancer therapeutic agent.
Comparison with Related Compounds
To understand the specificity and potency of this compound, it is valuable to compare it with similar compounds such as 1-(2-Furoyl)piperazine and 1-(4-Furoyl)piperazine.
Compound | Antimicrobial Activity | Anticancer Activity | Notes |
---|---|---|---|
This compound | High | Significant | Broad spectrum against Gram+ |
1-(2-Furoyl)piperazine | Moderate | Moderate | Different binding affinities |
1-(4-Furoyl)piperazine | Low | Minimal | Less effective than 3-position |
The position of the furoyl group significantly influences both the chemical reactivity and biological activity of these compounds.
Q & A
Q. What are the common synthetic routes for preparing 1-(3-Furoyl)piperazine derivatives, and how do reaction conditions influence yield and purity?
Level: Basic
Methodological Answer:
The synthesis of piperazine derivatives typically involves nucleophilic substitution or coupling reactions. For example, 1-(2-fluorobenzyl)piperazine analogs are synthesized via:
- Alkylation: Reacting 1-(2-fluorobenzyl)piperazine with propargyl bromide in dimethylformamide (DMF) using K₂CO₃ as a base, yielding intermediates with ~93% purity after silica gel chromatography .
- Click Chemistry: Copper-catalyzed azide-alkyne cycloaddition (CuAAC) with sodium ascorbate and CuSO₄·5H₂O in a water-DCM solvent system, producing triazole derivatives with yields up to 97% .
Optimization of reaction time, solvent polarity (e.g., hexane/ethyl acetate gradients), and stoichiometric ratios (e.g., 1.2 equiv. of azide derivatives) ensures high purity. Excess reagents and prolonged reaction times may lead to byproducts, necessitating TLC monitoring .
Q. How can Raman microspectroscopy parameters be optimized to differentiate between structurally similar piperazine analogs, including isomers?
Level: Advanced
Methodological Answer:
Raman microspectroscopy distinguishes isomers (e.g., 3-TFMPP vs. 4-TFMPP) using:
- Laser Power and Scans: 20 mW laser power with 128–256 scans enhances spectral resolution and intensity without degrading samples .
- Multivariate Analysis: Principal Component Analysis (PCA) reduces dimensionality, with PC2–PC4 explaining >99% variance for isomer groups. Linear Discriminant Analysis (LDA) of PCA scores achieves clear separation in LDA plots, validated by triplicate measurements .
Critical parameters include baseline correction and spectral normalization to minimize noise. This method is superior to GC-MS for non-destructive, in-situ analysis of crystalline or powdered samples .
Q. What analytical challenges arise in distinguishing positional isomers of halogenated phenylpiperazines, and which techniques are most effective?
Level: Advanced
Methodological Answer:
Positional isomers (e.g., 3-CPP vs. 4-CPP) pose challenges due to identical molecular weights and similar fragmentation patterns in MS. Effective strategies include:
- Chromatographic Separation: HPLC with phenyl-hexyl columns and methanol/ammonium formate gradients resolves isomers via subtle polarity differences .
- UV-Vis Spectroscopy: Derivatives like 1-(2-methoxyphenyl)piperazine exhibit distinct λmax shifts (e.g., 239 nm vs. 288 nm) for nitro or chloro substituents .
- NMR Spectroscopy: ¹H NMR coupling constants and ¹³C chemical shifts (e.g., δ 52.4–55.2 ppm for piperazine-CH₂ groups) differentiate ortho/meta/para substitution .
Q. What methodologies are employed to evaluate the biological activity of piperazine derivatives, particularly in receptor binding studies?
Level: Basic
Methodological Answer:
- Radioligand Binding Assays: Competitive displacement studies using ³H-labeled serotonin (5-HT) or dopamine (DA) receptors quantify IC₅₀ values. For example, 1-(3-chlorophenyl)piperazine (mCPP) shows affinity for 5-HT₂B/2C receptors (Kᵢ < 100 nM) .
- Molecular Docking: AutoDock Vina or Schrödinger Suite predicts binding poses, with scoring functions (e.g., Glide XP) validating interactions with residues like Asp155 in the 5-HT₂C active site .
- Functional Assays: Calcium flux or cAMP accumulation assays in HEK293 cells transfected with GPCRs measure agonist/antagonist efficacy .
Q. How do multivariate statistical analyses enhance the interpretation of spectroscopic data for piperazine analogs?
Level: Advanced
Methodological Answer:
Multivariate methods like PCA and LDA transform spectral data into interpretable models:
- PCA: Reduces 1500–2000 cm⁻¹ Raman spectra to 5 principal components (PCs), capturing >95% variance. For example, PC3 separates chlorophenyl isomers via C-Cl stretching modes at 650–750 cm⁻¹ .
- LDA: Classifies analogs into groups (e.g., benzylpiperazines vs. phenylpiperazines) using Mahalanobis distance, achieving >98% cross-validation accuracy .
Software tools (e.g., MATLAB PLS Toolbox) enable preprocessing (Savitzky-Golay smoothing) and outlier detection (Hotelling’s T²).
Q. What are the critical considerations in designing piperazine-based ligands for dopamine or serotonin receptors?
Level: Advanced
Methodological Answer:
- Substituent Effects: Electron-withdrawing groups (e.g., -CF₃ at meta positions) enhance 5-HT₂C affinity by 10-fold compared to -OCH₃ .
- Bioisosterism: Replacing phenyl with pyridyl rings improves metabolic stability (e.g., t₁/₂ > 2 hrs in liver microsomes) .
- Conformational Restriction: Introducing sp³-hybridized carbons (e.g., cyclohexenyl groups) reduces off-target binding to DAT .
Docking simulations must account for receptor flexibility, particularly in the orthosteric pocket of dopamine transporters .
Q. What role do substituent positions on the phenyl ring play in the pharmacokinetic properties of phenylpiperazine derivatives?
Level: Advanced
Methodological Answer:
- Meta-Substitution: 3-CPP exhibits higher BBB permeability (logBB = 0.8) than 4-CPP (logBB = 0.5) due to reduced polarity .
- Para-Substitution: 4-Fluorophenyl groups increase plasma protein binding (>90%) via hydrophobic interactions, reducing free drug concentration .
- Ortho-Substitution: Steric hindrance from 2-methyl groups slows CYP3A4-mediated N-dealkylation, prolonging half-life .
Quantitative Structure-Activity Relationship (QSAR) models using Hammett σ constants predict metabolic clearance rates .
Properties
IUPAC Name |
furan-3-yl(piperazin-1-yl)methanone | |
---|---|---|
Source | PubChem | |
URL | https://pubchem.ncbi.nlm.nih.gov | |
Description | Data deposited in or computed by PubChem | |
InChI |
InChI=1S/C9H12N2O2/c12-9(8-1-6-13-7-8)11-4-2-10-3-5-11/h1,6-7,10H,2-5H2 | |
Source | PubChem | |
URL | https://pubchem.ncbi.nlm.nih.gov | |
Description | Data deposited in or computed by PubChem | |
InChI Key |
PQYHMMSELUVQFX-UHFFFAOYSA-N | |
Source | PubChem | |
URL | https://pubchem.ncbi.nlm.nih.gov | |
Description | Data deposited in or computed by PubChem | |
Canonical SMILES |
C1CN(CCN1)C(=O)C2=COC=C2 | |
Source | PubChem | |
URL | https://pubchem.ncbi.nlm.nih.gov | |
Description | Data deposited in or computed by PubChem | |
Molecular Formula |
C9H12N2O2 | |
Source | PubChem | |
URL | https://pubchem.ncbi.nlm.nih.gov | |
Description | Data deposited in or computed by PubChem | |
DSSTOX Substance ID |
DTXSID60585500 | |
Record name | (Furan-3-yl)(piperazin-1-yl)methanone | |
Source | EPA DSSTox | |
URL | https://comptox.epa.gov/dashboard/DTXSID60585500 | |
Description | DSSTox provides a high quality public chemistry resource for supporting improved predictive toxicology. | |
Molecular Weight |
180.20 g/mol | |
Source | PubChem | |
URL | https://pubchem.ncbi.nlm.nih.gov | |
Description | Data deposited in or computed by PubChem | |
CAS No. |
885326-62-5 | |
Record name | (Furan-3-yl)(piperazin-1-yl)methanone | |
Source | EPA DSSTox | |
URL | https://comptox.epa.gov/dashboard/DTXSID60585500 | |
Description | DSSTox provides a high quality public chemistry resource for supporting improved predictive toxicology. | |
Disclaimer and Information on In-Vitro Research Products
Please be aware that all articles and product information presented on BenchChem are intended solely for informational purposes. The products available for purchase on BenchChem are specifically designed for in-vitro studies, which are conducted outside of living organisms. In-vitro studies, derived from the Latin term "in glass," involve experiments performed in controlled laboratory settings using cells or tissues. It is important to note that these products are not categorized as medicines or drugs, and they have not received approval from the FDA for the prevention, treatment, or cure of any medical condition, ailment, or disease. We must emphasize that any form of bodily introduction of these products into humans or animals is strictly prohibited by law. It is essential to adhere to these guidelines to ensure compliance with legal and ethical standards in research and experimentation.