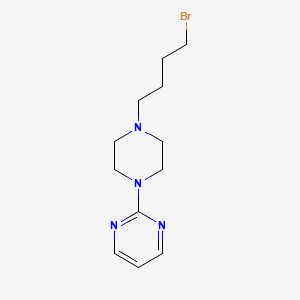
2-(4-(4-Bromobutyl)piperazin-1-yl)pyrimidine
Overview
Description
“2-(4-(4-Bromobutyl)piperazin-1-yl)pyrimidine” is a compound that contains a pyrimidine ring linked to a piperazine ring via a 4-bromobutyl chain . It is a part of a class of compounds known as N-arylpiperazines . Piperazine is a common structural motif found in agrochemicals and pharmaceuticals, in part due to its ability to positively modulate the pharmacokinetic properties of a drug substance .
Synthesis Analysis
The synthesis of similar compounds has been reported in the literature. For instance, a novel compound “2- { [4- (4-bromophenyl)piperazin-1-yl)]methyl}-4- (3-chlorophenyl)-5- (4-methoxyphenyl)-2,4-dihydro-3H-1,2,4-triazole-3-thione” was obtained in good yield via a three-step protocol . The reaction with amine was carried out in 95% ethanol at room temperature for 24 hours .
Physical And Chemical Properties Analysis
The physical and chemical properties of “this compound” include a density of 1.3±0.1 g/cm³, boiling point of 418.0±55.0 °C at 760 mmHg, vapour pressure of 0.0±1.0 mmHg at 25°C, enthalpy of vaporization of 67.2±3.0 kJ/mol, flash point of 206.6±31.5 °C, index of refraction of 1.564, molar refractivity of 72.5±0.3 cm³, and a molar volume of 223.0±3.0 cm³ .
Scientific Research Applications
Synthesis and Pharmacological Potential
Synthesis of New Derivatives :The compound has been utilized in the synthesis of hexahydro- and octahydropyrido[1,2-c]pyrimidine derivatives with an arylpiperazine moiety, targeting the affinity towards 5-HT1A, 5-HT2A, and alpha1 adrenergic receptors. These derivatives are synthesized through multi-stage techniques, starting from 4-arylhexahydro-1H,3H-pyrido[1,2-c]pyrimidine-1,3-dione derivatives and undergoing N-alkylation with 1,4-dibromobutane to yield bromobutyl derivatives. The final products show potential for pharmacological investigation (Herold, Król, & Kleps, 2004).
Antiproliferative Activity :2-Methyl-3-(2-piperazin-1-yl-ethyl)-pyrido[1,2-a]pyrimidin-4-one derivatives have been synthesized and evaluated for antiproliferative activity against human cancer cell lines. These compounds, characterized by spectral studies, show moderate to good activity, indicating potential as anticancer agents. Among the series, certain derivatives exhibit significant inhibitory activities, suggesting the need for further research (Mallesha et al., 2012).
Antimicrobial Activity
Dithiocarbamate Derivatives :New dithiocarbamate derivatives bearing thiazole/benzothiazole rings have been synthesized, starting from the potassium salt of 4-(2-pyrimidinyl)piperazine dithiocarbamate. These compounds exhibit high antimicrobial activity against various microorganism strains, highlighting their potential in antimicrobial applications (Yurttaş et al., 2016).
Molecular Biology Applications
Tyrosine Kinase Inhibitor Synthesis :Research on the synthesis of PD0205520, a tyrosine kinase inhibitor potentially useful in cancer treatment, involves the use of pyrimidine derivatives. These compounds, labeled with radio- and stable-isotopes, are crucial for studying drug absorption, distribution, metabolism, and excretion, illustrating the compound's role in developing targeted cancer therapies (Zhang, Huang, & Huang, 2005).
Mechanism of Action
Target of Action
Similar compounds with a piperazine moiety have been found to interact with a variety of targets, such as acetylcholinesterase (ache) and butyrylcholinesterase (buche) . These enzymes play crucial roles in the nervous system, particularly in the hydrolysis of the neurotransmitter acetylcholine .
Mode of Action
Compounds with similar structures have been shown to inhibit ache and buche . Inhibition of these enzymes increases the concentration of acetylcholine in the synaptic cleft, enhancing cholinergic neurotransmission .
Biochemical Pathways
Inhibition of ache and buche, as seen with similar compounds, would affect the cholinergic pathway . This could lead to enhanced cholinergic neurotransmission, potentially affecting cognitive function.
Pharmacokinetics
The piperazine moiety is known to positively modulate the pharmacokinetic properties of a drug substance . This could potentially enhance the absorption, distribution, metabolism, and excretion (ADME) properties of the compound, thereby improving its bioavailability .
Result of Action
Similar compounds have been shown to inhibit ache and buche , which could potentially lead to enhanced cholinergic neurotransmission and affect cognitive function.
Biochemical Analysis
Biochemical Properties
2-(4-(4-Bromobutyl)piperazin-1-yl)pyrimidine plays a significant role in biochemical reactions due to its ability to interact with enzymes, proteins, and other biomolecules. The compound has been shown to bind to specific enzymes, potentially inhibiting or activating their activity. For example, it may interact with kinases, which are enzymes that play a crucial role in cell signaling pathways. The nature of these interactions can vary, with this compound acting as either an inhibitor or an activator, depending on the specific enzyme and the context of the reaction .
Cellular Effects
The effects of this compound on various types of cells and cellular processes are diverse. This compound can influence cell function by modulating cell signaling pathways, gene expression, and cellular metabolism. For instance, it may affect the phosphorylation status of proteins involved in signaling cascades, thereby altering the downstream effects on gene expression and metabolic pathways. Studies have shown that this compound can impact cell viability, proliferation, and apoptosis in different cell types .
Molecular Mechanism
At the molecular level, this compound exerts its effects through specific binding interactions with biomolecules. The compound can bind to the active sites of enzymes, leading to inhibition or activation of their catalytic activity. Additionally, it may interact with transcription factors, influencing gene expression by modulating their binding to DNA. These molecular interactions are crucial for understanding the compound’s mechanism of action and its potential therapeutic applications .
Temporal Effects in Laboratory Settings
In laboratory settings, the effects of this compound can change over time. The stability and degradation of the compound are important factors to consider, as they can influence its long-term effects on cellular function. Studies have shown that the compound remains stable under certain conditions, but may degrade over time, leading to a decrease in its efficacy. Long-term exposure to this compound can result in changes in cellular function, including alterations in gene expression and metabolic activity .
Dosage Effects in Animal Models
The effects of this compound vary with different dosages in animal models. At lower doses, the compound may exhibit beneficial effects, such as enhancing cell viability and function. At higher doses, it can become toxic, leading to adverse effects such as cell death and tissue damage. Threshold effects have been observed, where a specific dosage range is required to achieve the desired therapeutic effect without causing toxicity .
Metabolic Pathways
This compound is involved in various metabolic pathways, interacting with enzymes and cofactors that regulate metabolic flux and metabolite levels. The compound can influence the activity of enzymes involved in key metabolic processes, such as glycolysis and the citric acid cycle. By modulating these pathways, this compound can alter the overall metabolic state of cells, impacting their energy production and biosynthetic capabilities .
Transport and Distribution
The transport and distribution of this compound within cells and tissues are mediated by specific transporters and binding proteins. These interactions determine the localization and accumulation of the compound in different cellular compartments. The compound’s distribution can affect its activity and function, as it may need to reach specific sites within the cell to exert its effects .
Subcellular Localization
The subcellular localization of this compound is crucial for its activity and function. The compound may be directed to specific compartments or organelles through targeting signals or post-translational modifications. For example, it may localize to the nucleus to interact with transcription factors or to the mitochondria to influence metabolic processes. Understanding the subcellular localization of this compound is essential for elucidating its mechanism of action and potential therapeutic applications.
properties
IUPAC Name |
2-[4-(4-bromobutyl)piperazin-1-yl]pyrimidine | |
---|---|---|
Source | PubChem | |
URL | https://pubchem.ncbi.nlm.nih.gov | |
Description | Data deposited in or computed by PubChem | |
InChI |
InChI=1S/C12H19BrN4/c13-4-1-2-7-16-8-10-17(11-9-16)12-14-5-3-6-15-12/h3,5-6H,1-2,4,7-11H2 | |
Source | PubChem | |
URL | https://pubchem.ncbi.nlm.nih.gov | |
Description | Data deposited in or computed by PubChem | |
InChI Key |
PSBMPSNKIDFXIK-UHFFFAOYSA-N | |
Source | PubChem | |
URL | https://pubchem.ncbi.nlm.nih.gov | |
Description | Data deposited in or computed by PubChem | |
Canonical SMILES |
C1CN(CCN1CCCCBr)C2=NC=CC=N2 | |
Source | PubChem | |
URL | https://pubchem.ncbi.nlm.nih.gov | |
Description | Data deposited in or computed by PubChem | |
Molecular Formula |
C12H19BrN4 | |
Source | PubChem | |
URL | https://pubchem.ncbi.nlm.nih.gov | |
Description | Data deposited in or computed by PubChem | |
DSSTOX Substance ID |
DTXSID101259312 | |
Record name | 2-[4-(4-Bromobutyl)-1-piperazinyl]pyrimidine | |
Source | EPA DSSTox | |
URL | https://comptox.epa.gov/dashboard/DTXSID101259312 | |
Description | DSSTox provides a high quality public chemistry resource for supporting improved predictive toxicology. | |
Molecular Weight |
299.21 g/mol | |
Source | PubChem | |
URL | https://pubchem.ncbi.nlm.nih.gov | |
Description | Data deposited in or computed by PubChem | |
CAS RN |
87789-48-8 | |
Record name | 2-[4-(4-Bromobutyl)-1-piperazinyl]pyrimidine | |
Source | CAS Common Chemistry | |
URL | https://commonchemistry.cas.org/detail?cas_rn=87789-48-8 | |
Description | CAS Common Chemistry is an open community resource for accessing chemical information. Nearly 500,000 chemical substances from CAS REGISTRY cover areas of community interest, including common and frequently regulated chemicals, and those relevant to high school and undergraduate chemistry classes. This chemical information, curated by our expert scientists, is provided in alignment with our mission as a division of the American Chemical Society. | |
Explanation | The data from CAS Common Chemistry is provided under a CC-BY-NC 4.0 license, unless otherwise stated. | |
Record name | 2-[4-(4-Bromobutyl)-1-piperazinyl]pyrimidine | |
Source | EPA DSSTox | |
URL | https://comptox.epa.gov/dashboard/DTXSID101259312 | |
Description | DSSTox provides a high quality public chemistry resource for supporting improved predictive toxicology. | |
Disclaimer and Information on In-Vitro Research Products
Please be aware that all articles and product information presented on BenchChem are intended solely for informational purposes. The products available for purchase on BenchChem are specifically designed for in-vitro studies, which are conducted outside of living organisms. In-vitro studies, derived from the Latin term "in glass," involve experiments performed in controlled laboratory settings using cells or tissues. It is important to note that these products are not categorized as medicines or drugs, and they have not received approval from the FDA for the prevention, treatment, or cure of any medical condition, ailment, or disease. We must emphasize that any form of bodily introduction of these products into humans or animals is strictly prohibited by law. It is essential to adhere to these guidelines to ensure compliance with legal and ethical standards in research and experimentation.