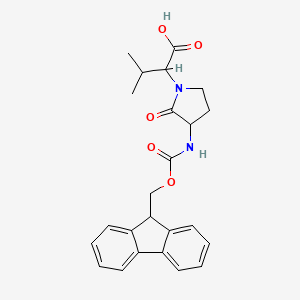
N-Fmoc-Freidinger's lactam
- Click on QUICK INQUIRY to receive a quote from our team of experts.
- With the quality product at a COMPETITIVE price, you can focus more on your research.
Overview
Description
Preparation Methods
Synthetic Routes and Reaction Conditions
N-Fmoc-Freidinger’s lactam can be synthesized through a series of chemical reactions involving the cyclization of amino acids. One common method involves the use of a methionine sulfonium salt to induce cyclization, resulting in the formation of the lactam ring . The reaction conditions typically include the use of organic solvents and catalysts to facilitate the cyclization process.
Industrial Production Methods
Industrial production of N-Fmoc-Freidinger’s lactam involves large-scale synthesis using automated peptide synthesizers. These machines allow for precise control over reaction conditions, ensuring high yields and purity of the final product. The process often includes multiple purification steps, such as chromatography, to remove impurities and obtain the desired compound .
Chemical Reactions Analysis
Types of Reactions
N-Fmoc-Freidinger’s lactam undergoes various chemical reactions, including:
Oxidation: The compound can be oxidized using oxidizing agents such as hydrogen peroxide or potassium permanganate.
Reduction: Reduction reactions can be carried out using reducing agents like sodium borohydride or lithium aluminum hydride.
Substitution: N-Fmoc-Freidinger’s lactam can undergo substitution reactions with nucleophiles, such as amines or thiols, to form new derivatives.
Common Reagents and Conditions
Oxidation: Hydrogen peroxide, potassium permanganate; typically carried out in aqueous or organic solvents.
Reduction: Sodium borohydride, lithium aluminum hydride; reactions are often performed in anhydrous conditions to prevent side reactions.
Substitution: Amines, thiols; reactions are usually conducted in the presence of a base to facilitate nucleophilic attack.
Major Products Formed
The major products formed from these reactions include various derivatives of N-Fmoc-Freidinger’s lactam, which can be used in further chemical synthesis or as intermediates in the production of more complex molecules .
Scientific Research Applications
N-Fmoc-Freidinger’s lactam has a wide range of applications in scientific research, including:
Chemistry: Used as a building block in the synthesis of complex peptides and peptidomimetics.
Biology: Employed in the study of protein-protein interactions and enzyme mechanisms.
Medicine: Investigated for its potential therapeutic applications, such as in the development of peptide-based drugs.
Industry: Utilized in the production of high-purity peptides for research and pharmaceutical purposes.
Mechanism of Action
The mechanism of action of N-Fmoc-Freidinger’s lactam involves its ability to mimic the structure of natural peptides. By adopting a similar conformation, it can interact with biological targets, such as enzymes and receptors, in a manner similar to natural peptides. This allows it to modulate biological pathways and exert its effects .
Comparison with Similar Compounds
Similar Compounds
Beta-lactams: A class of antibiotics that includes penicillins and cephalosporins, which also contain a lactam ring.
Gamma-lactams: Compounds with a five-membered lactam ring, used in various pharmaceutical applications.
Delta-lactams: Six-membered lactam rings, often found in natural products and synthetic drugs.
Uniqueness
N-Fmoc-Freidinger’s lactam is unique due to its conformational restriction, which provides enhanced stability and resistance to enzymatic degradation compared to other lactams. This makes it particularly valuable in peptide synthesis and drug development .
Properties
CAS No. |
957507-85-6 |
---|---|
Molecular Formula |
C24H26N2O5 |
Molecular Weight |
422.5 g/mol |
IUPAC Name |
2-[3-(9H-fluoren-9-ylmethoxycarbonylamino)-2-oxopyrrolidin-1-yl]-3-methylbutanoic acid |
InChI |
InChI=1S/C24H26N2O5/c1-14(2)21(23(28)29)26-12-11-20(22(26)27)25-24(30)31-13-19-17-9-5-3-7-15(17)16-8-4-6-10-18(16)19/h3-10,14,19-21H,11-13H2,1-2H3,(H,25,30)(H,28,29) |
InChI Key |
PEMHOAIHPQJNIM-UHFFFAOYSA-N |
Canonical SMILES |
CC(C)C(C(=O)O)N1CCC(C1=O)NC(=O)OCC2C3=CC=CC=C3C4=CC=CC=C24 |
Origin of Product |
United States |
Disclaimer and Information on In-Vitro Research Products
Please be aware that all articles and product information presented on BenchChem are intended solely for informational purposes. The products available for purchase on BenchChem are specifically designed for in-vitro studies, which are conducted outside of living organisms. In-vitro studies, derived from the Latin term "in glass," involve experiments performed in controlled laboratory settings using cells or tissues. It is important to note that these products are not categorized as medicines or drugs, and they have not received approval from the FDA for the prevention, treatment, or cure of any medical condition, ailment, or disease. We must emphasize that any form of bodily introduction of these products into humans or animals is strictly prohibited by law. It is essential to adhere to these guidelines to ensure compliance with legal and ethical standards in research and experimentation.