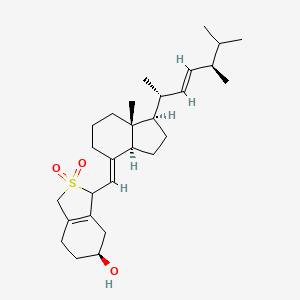
Vitamin D2 SO2 Adduct
- Click on QUICK INQUIRY to receive a quote from our team of experts.
- With the quality product at a COMPETITIVE price, you can focus more on your research.
Overview
Description
Vitamin D2 SO2 Adduct is a chemical compound formed by the reaction of Vitamin D2 (ergocalciferol) with sulfur dioxideThe adduct formation involves the addition of sulfur dioxide to the Δ5,10(19)-diene part of Vitamin D2, resulting in the formation of α and β-face adducts in approximately equal ratios .
Preparation Methods
Synthetic Routes and Reaction Conditions
The synthesis of Vitamin D2 SO2 Adduct involves the reaction of Vitamin D2 with liquid sulfur dioxide. The reaction occurs rapidly at the Δ5,10(19)-diene part of Vitamin D2, leading to the formation of α and β-face adducts . The reaction conditions typically involve refluxing the mixture for about 30 minutes .
Industrial Production Methods
While specific industrial production methods for this compound are not well-documented, the general approach would involve scaling up the laboratory synthesis process. This would include the use of larger reaction vessels, controlled addition of sulfur dioxide, and efficient cooling systems to manage the exothermic nature of the reaction.
Chemical Reactions Analysis
Types of Reactions
Vitamin D2 SO2 Adduct undergoes several types of chemical reactions, including:
Oxidation and Reduction: The compound can participate in oxidation and reduction reactions, although specific details on these reactions are limited.
Common Reagents and Conditions
Common reagents used in the reactions involving this compound include sulfur dioxide for the initial adduct formation and heat for the thermolysis process. The reaction conditions typically involve refluxing the mixture and controlled heating to achieve the desired products .
Major Products Formed
The major products formed from the reactions of this compound include 5,6-trans Vitamin D2 and a trace amount of Vitamin D2 .
Scientific Research Applications
Vitamin D2 SO2 Adduct has several scientific research applications, including:
Chemistry: The compound is used to study the reactivity and stability of Vitamin D derivatives. It serves as a model compound for understanding the behavior of similar adducts.
Biology: Research on this compound helps in understanding the biological activity of Vitamin D derivatives and their potential therapeutic applications.
Medicine: The compound’s unique properties make it a candidate for developing new drugs and treatments, particularly in the field of bone health and calcium metabolism.
Industry: This compound can be used in the development of fortified foods and supplements, leveraging its stability and bioavailability
Mechanism of Action
The mechanism of action of Vitamin D2 SO2 Adduct involves its interaction with molecular targets such as the Vitamin D receptor (VDR). Upon binding to VDR, the adduct can modulate the transcription of genes involved in calcium and phosphate homeostasis, bone mineral remodeling, and immune function . The adduct’s effects are mediated through the formation of a heterodimer with the retinoid X receptor (RXR), which then binds to Vitamin D responsive elements in the DNA .
Comparison with Similar Compounds
Similar Compounds
Vitamin D2 (Ergocalciferol): The parent compound of Vitamin D2 SO2 Adduct, primarily found in fungi and mushrooms.
Vitamin D3 (Cholecalciferol): Another form of Vitamin D, synthesized in the skin upon exposure to sunlight and found in animal-sourced foods
Uniqueness
This compound is unique due to its formation through the addition of sulfur dioxide, which imparts distinct chemical properties and reactivity compared to its parent compound, Vitamin D2. This uniqueness makes it valuable for specific research applications and potential therapeutic uses .
Properties
Molecular Formula |
C28H44O3S |
---|---|
Molecular Weight |
460.7 g/mol |
IUPAC Name |
(5S)-3-[(E)-[(1R,3aS,7aR)-1-[(E,2R,5R)-5,6-dimethylhept-3-en-2-yl]-7a-methyl-2,3,3a,5,6,7-hexahydro-1H-inden-4-ylidene]methyl]-2,2-dioxo-1,3,4,5,6,7-hexahydro-2-benzothiophen-5-ol |
InChI |
InChI=1S/C28H44O3S/c1-18(2)19(3)8-9-20(4)25-12-13-26-21(7-6-14-28(25,26)5)15-27-24-16-23(29)11-10-22(24)17-32(27,30)31/h8-9,15,18-20,23,25-27,29H,6-7,10-14,16-17H2,1-5H3/b9-8+,21-15+/t19-,20+,23-,25+,26-,27?,28+/m0/s1 |
InChI Key |
LYTLCKCMCADGOA-OXNYQFQASA-N |
Isomeric SMILES |
C[C@H](/C=C/[C@H](C)C(C)C)[C@H]1CC[C@@H]\2[C@@]1(CCC/C2=C\C3C4=C(CC[C@@H](C4)O)CS3(=O)=O)C |
Canonical SMILES |
CC(C)C(C)C=CC(C)C1CCC2C1(CCCC2=CC3C4=C(CCC(C4)O)CS3(=O)=O)C |
Origin of Product |
United States |
Disclaimer and Information on In-Vitro Research Products
Please be aware that all articles and product information presented on BenchChem are intended solely for informational purposes. The products available for purchase on BenchChem are specifically designed for in-vitro studies, which are conducted outside of living organisms. In-vitro studies, derived from the Latin term "in glass," involve experiments performed in controlled laboratory settings using cells or tissues. It is important to note that these products are not categorized as medicines or drugs, and they have not received approval from the FDA for the prevention, treatment, or cure of any medical condition, ailment, or disease. We must emphasize that any form of bodily introduction of these products into humans or animals is strictly prohibited by law. It is essential to adhere to these guidelines to ensure compliance with legal and ethical standards in research and experimentation.