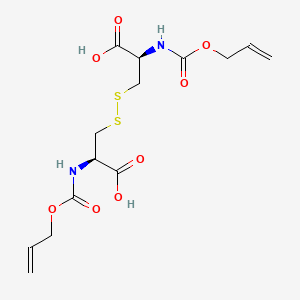
Alloc-Cys(1)-OH.Alloc-Cys(1)-OH
- Click on QUICK INQUIRY to receive a quote from our team of experts.
- With the quality product at a COMPETITIVE price, you can focus more on your research.
Overview
Description
Alloc-Cys(1)-OH.Alloc-Cys(1)-OH, also known as N-allyloxycarbonyl-L-cysteine, is a derivative of the amino acid cysteine. This compound is primarily used in peptide synthesis as a protecting group for the thiol group of cysteine. Protecting groups are essential in synthetic chemistry to prevent unwanted reactions at specific functional groups during multi-step synthesis processes .
Preparation Methods
Synthetic Routes and Reaction Conditions
Alloc-Cys(1)-OH.Alloc-Cys(1)-OH can be synthesized through various methods. One common approach involves the protection of the cysteine thiol group using allyloxycarbonyl (Alloc) groups. The reaction typically involves the use of allyl chloroformate and a base such as triethylamine to form the Alloc-protected cysteine .
Industrial Production Methods
Industrial production of this compound follows similar synthetic routes but on a larger scale. The process involves optimizing reaction conditions to ensure high yields and purity. The use of automated synthesis equipment and continuous flow reactors can enhance efficiency and scalability .
Chemical Reactions Analysis
Types of Reactions
Alloc-Cys(1)-OH.Alloc-Cys(1)-OH undergoes various chemical reactions, including:
Deprotection Reactions: The Alloc group can be removed under mild conditions using palladium catalysts, such as tetrakis(triphenylphosphine)palladium(0), in the presence of a nucleophile like morpholine.
Amidation Reactions: The compound can react with carboxylic acids or their derivatives to form amides.
Common Reagents and Conditions
Deprotection: Palladium catalysts and nucleophiles (e.g., morpholine).
Amidation: Carbodiimides and carboxylic acid derivatives.
Major Products Formed
Deprotection: Free cysteine.
Amidation: Amide derivatives of cysteine.
Scientific Research Applications
Alloc-Cys(1)-OH.Alloc-Cys(1)-OH has numerous applications in scientific research:
Peptide Synthesis: Used as a protecting group for cysteine in solid-phase peptide synthesis, facilitating the synthesis of complex peptides and proteins.
Protein Modification: Employed in the site-specific modification of proteins, enabling the study of protein function and interactions.
Drug Development: Utilized in the synthesis of peptide-based drugs and therapeutic agents.
Bioconjugation: Applied in the conjugation of peptides to other biomolecules, such as antibodies, for targeted drug delivery and diagnostics.
Mechanism of Action
The primary mechanism of action of Alloc-Cys(1)-OH.Alloc-Cys(1)-OH involves the protection and subsequent deprotection of the cysteine thiol group. The Alloc group prevents unwanted reactions at the thiol group during synthesis. Upon deprotection, the free thiol group can participate in various biochemical reactions, such as disulfide bond formation and nucleophilic substitution .
Comparison with Similar Compounds
Alloc-Cys(1)-OH.Alloc-Cys(1)-OH is unique compared to other cysteine derivatives due to its specific protecting group. Similar compounds include:
Boc-Cys(1)-OH: Uses a tert-butoxycarbonyl (Boc) protecting group.
Cbz-Cys(1)-OH: Uses a benzyloxycarbonyl (Cbz) protecting group.
This compound offers advantages in terms of mild deprotection conditions and compatibility with various synthetic methods, making it a valuable tool in peptide and protein chemistry .
Properties
Molecular Formula |
C14H20N2O8S2 |
---|---|
Molecular Weight |
408.5 g/mol |
IUPAC Name |
(2R)-3-[[(2R)-2-carboxy-2-(prop-2-enoxycarbonylamino)ethyl]disulfanyl]-2-(prop-2-enoxycarbonylamino)propanoic acid |
InChI |
InChI=1S/C14H20N2O8S2/c1-3-5-23-13(21)15-9(11(17)18)7-25-26-8-10(12(19)20)16-14(22)24-6-4-2/h3-4,9-10H,1-2,5-8H2,(H,15,21)(H,16,22)(H,17,18)(H,19,20)/t9-,10-/m0/s1 |
InChI Key |
SWVORQKGWDILLE-UWVGGRQHSA-N |
Isomeric SMILES |
C=CCOC(=O)N[C@@H](CSSC[C@@H](C(=O)O)NC(=O)OCC=C)C(=O)O |
Canonical SMILES |
C=CCOC(=O)NC(CSSCC(C(=O)O)NC(=O)OCC=C)C(=O)O |
Origin of Product |
United States |
Disclaimer and Information on In-Vitro Research Products
Please be aware that all articles and product information presented on BenchChem are intended solely for informational purposes. The products available for purchase on BenchChem are specifically designed for in-vitro studies, which are conducted outside of living organisms. In-vitro studies, derived from the Latin term "in glass," involve experiments performed in controlled laboratory settings using cells or tissues. It is important to note that these products are not categorized as medicines or drugs, and they have not received approval from the FDA for the prevention, treatment, or cure of any medical condition, ailment, or disease. We must emphasize that any form of bodily introduction of these products into humans or animals is strictly prohibited by law. It is essential to adhere to these guidelines to ensure compliance with legal and ethical standards in research and experimentation.