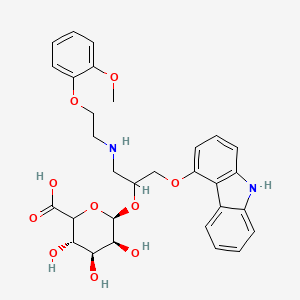
Carvedilol beta-D-Glucuronide (mixture of diasteromers)
- Click on QUICK INQUIRY to receive a quote from our team of experts.
- With the quality product at a COMPETITIVE price, you can focus more on your research.
Overview
Description
Carvedilol β-D-glucuronide is a major phase II metabolite of carvedilol, a nonselective β-adrenergic and α1-adrenergic receptor blocker used to treat hypertension and heart failure . The glucuronidation occurs at the hydroxyl group of the carbazole moiety, resulting in a mixture of diastereomers due to stereochemical variability at the glucuronide linkage site . Its molecular formula is C30H34N2O10, with a molecular weight of 582.6 g/mol . As a metabolite, it is pharmacologically inactive compared to the parent drug but plays a critical role in carvedilol’s elimination pathway .
Preparation Methods
Synthetic Routes and Reaction Conditions: The synthesis of Carvedilol beta-D-Glucuronide involves the enzymatic or chemical conjugation of Carvedilol with glucuronic acid. The reaction typically occurs in the presence of uridine 5’-diphospho-glucuronosyltransferase (UGT) enzymes . The reaction conditions often include a buffered aqueous solution at physiological pH and temperature to facilitate the enzymatic activity.
Industrial Production Methods: Industrial production of Carvedilol beta-D-Glucuronide may involve biotransformation processes using microbial or mammalian cell cultures expressing UGT enzymes. This method ensures high yield and purity of the glucuronide conjugate .
Chemical Reactions Analysis
CYP3A Inhibition
Carvedilol β-D-glucuronide exhibits weak time-dependent inhibition of CYP3A , but the parent drug carvedilol is a more potent inhibitor. Preincubation with NADPH reduces carvedilol’s IC₅₀ for CYP3A inhibition from 7.0 µM to 1.1 µM, with a k<sub>inact</sub> of 0.051/min and K<sub>I</sub> of 1.8 µM . This interaction elevates plasma concentrations of CYP3A substrates (e.g., midazolam, simvastatin), necessitating dose adjustments in combination therapies.
CYP3A Inhibition Parameters
Parameter | Value | Source |
---|---|---|
IC₅₀ (preincubated) | 1.1 µM | |
k<sub>inact</sub> | 0.051/min | |
K<sub>I</sub> | 1.8 µM |
Enantiomer-Specific Glucuronidation
The racemic nature of carvedilol influences glucuronidation rates:
-
S-carvedilol undergoes faster glucuronidation (0.51 pmol/min/mg protein) compared to R-carvedilol (0.026 pmol/min/mg protein) .
-
Amiodarone enhances glucuronidation of R-carvedilol by 6.15-fold and S-carvedilol by 1.60-fold in human liver microsomes (HLM) with bovine serum albumin (BSA) .
Effect of Amiodarone on Glucuronidation
Enantiomer | Rate (pmol/min/mg protein) | Fold Increase (with 50 µM Amiodarone) |
---|---|---|
R-carvedilol | 0.026 | 6.15 |
S-carvedilol | 0.51 | 1.60 |
Stereochemical Considerations
-
R- vs. S-enantiomers : While both contribute to α<sub>1</sub>-adrenergic blockade, only S-carvedilol exhibits β-blockade . This stereoselectivity influences the glucuronide’s pharmacological activity.
-
Diastereomer Formation : Glucuronidation introduces stereogenic centers, leading to diastereomeric mixtures. For example, carvedilol N′-β-D-glucuronide exists as distinct diastereomers with varying chromatographic retention times .
Analytical Differentiation
The deprotonation reaction distinguishes O- and N-glucuronides. For instance, deprotonated carvedilol N′-β-D-glucuronide forms a diagnostic product ion ([M − H]⁻ → [M − H − 162]⁻), aiding in structural identification .
Clinical Implications
-
Drug-Drug Interactions : Carvedilol’s CYP3A inhibition increases exposure to midazolam and simvastatin, requiring therapeutic monitoring .
-
Enantiomer-Specific Pharmacokinetics : The preferential glucuronidation of S-carvedilol may affect its efficacy in β-blockade-dependent conditions .
-
Metabolite Stability : Carvedilol β-D-glucuronide is primarily excreted in bile/feces, with minimal renal elimination .
Scientific Research Applications
Carvedilol beta-D-Glucuronide has several scientific research applications:
Mechanism of Action
Carvedilol beta-D-Glucuronide exerts its effects primarily through its parent compound, Carvedilol. Carvedilol acts by blocking beta-adrenergic receptors, reducing heart rate and blood pressure . The glucuronide conjugate may also interact with transport proteins, influencing its distribution and excretion in the body .
Comparison with Similar Compounds
Comparison with Other Carvedilol Metabolites and Related Compounds
Table 1: Key Carvedilol Metabolites and Impurities
Key Differences :
- Activity : Unlike carvedilol, its glucuronides lack β/α-blocking activity, emphasizing their role in detoxification .
- Synthesis : β-D-glucuronide is a major metabolite, while N-glucuronide is less prevalent and structurally distinct .
- Diastereomerism : The β-D-glucuronide exists as diastereomers, whereas N-glucuronide lacks this stereochemical complexity .
Comparison with Glucuronides of Other Drugs
Table 2: Structural and Functional Comparison with Other Drug Glucuronides
Key Insights :
- Activity Retention : Resveratrol glucuronide retains partial bioactivity, unlike carvedilol’s metabolite, which is fully inactivated .
- Analytical Methods : Carvedilol glucuronide is quantified via LC-MS, whereas fluorescent assays (e.g., 4-methylumbelliferyl glucuronide) are used for generic β-D-glucuronide detection .
Pharmacokinetic and Formulation Considerations
Carvedilol’s release from hypromellose matrix tablets is influenced by excipients (e.g., sucrose, maltodextrin), which modulate dissolution rates and subsequent glucuronidation . Mathematical models (e.g., Hopfenberg with Tlag) predict carvedilol release profiles but overestimate burst release, highlighting formulation challenges in controlling metabolite generation .
Table 3: Pharmacokinetic Parameters of Carvedilol and Metabolites
Parameter | Carvedilol | Carvedilol β-D-glucuronide |
---|---|---|
Half-life (t½) | 6–10 hours | Not reported |
Plasma protein binding | 95–98% | Likely lower |
Excretion pathway | Hepatic (CYP2D6) | Renal |
Bioactivity | Active (β/α-blocker) | Inactive |
Clinical Relevance : Carvedilol’s efficacy in reducing heart failure mortality (65% risk reduction) depends on maintaining therapeutic plasma levels, indirectly affected by glucuronide formation rates .
Biological Activity
Carvedilol beta-D-glucuronide is a significant metabolite of carvedilol, a third-generation non-selective beta-adrenergic antagonist and alpha-1 blocker. This article explores the biological activity of carvedilol beta-D-glucuronide, emphasizing its pharmacodynamics, pharmacokinetics, and implications in therapeutic contexts.
Overview of Carvedilol
Carvedilol is primarily used for treating chronic heart failure, hypertension, and left ventricular dysfunction. Its mechanism involves blocking beta-adrenergic receptors (β1 and β2) and alpha-1 adrenergic receptors, leading to decreased heart rate and vasodilation. The compound is known for its antioxidant properties and favorable metabolic profile compared to other beta-blockers, as it does not induce insulin resistance or negatively affect glucose metabolism .
Metabolism of Carvedilol
Carvedilol undergoes extensive first-pass metabolism, with approximately 25% to 35% bioavailability. It is primarily metabolized by liver enzymes (CYP2D6 and CYP2C9) through oxidation and glucuronidation, resulting in several active metabolites, including carvedilol beta-D-glucuronide. This metabolite retains some biological activity but exhibits a reduced potency compared to the parent compound .
Pharmacodynamics
The pharmacodynamic profile of carvedilol beta-D-glucuronide includes:
- Beta-Adrenergic Receptor Interaction : While carvedilol itself shows high affinity for β1 and β2 receptors (K_i values of 0.24–0.43 nM and 0.19–0.25 nM respectively), the glucuronide form exhibits diminished receptor activity. However, it may still contribute to the overall therapeutic effects of carvedilol through its influence on receptor signaling pathways .
- Biased Agonism : Research indicates that carvedilol acts as a biased agonist at β-adrenoceptors, engaging β-arrestin pathways that lead to beneficial metabolic effects without the adverse effects typically associated with other β-blockers. This mechanism may be partially retained in its glucuronide form .
Pharmacokinetics
The pharmacokinetic characteristics of carvedilol beta-D-glucuronide include:
- Absorption and Distribution : The glucuronide metabolite is likely to have altered absorption characteristics due to its polar nature compared to the lipophilic parent compound. It is expected to have a different volume of distribution and protein binding profile .
- Elimination : Carvedilol beta-D-glucuronide is primarily excreted via urine and bile, with limited excretion as unchanged drug. Its half-life is expected to be similar to that of carvedilol, ranging from 7 to 10 hours .
Case Studies and Research Findings
Several studies have investigated the effects of carvedilol and its metabolites:
- Diabetes-Induced Cardiac Dysfunction : In a study involving streptozotocin-induced diabetic rats, carvedilol treatment improved cardiac function by enhancing β-arrestin signaling pathways. Although specific data on the glucuronide metabolite were not detailed, the findings suggest that metabolites like carvedilol beta-D-glucuronide may play a role in these mechanisms .
- Bile Acid Homeostasis : Research indicates that carvedilol affects bile acid metabolism by downregulating transporters involved in bile acid uptake in hepatocytes. While this study focused on the parent compound, similar effects could be hypothesized for its glucuronide form due to shared metabolic pathways .
- Stereoselective Glucuronidation : A study highlighted the stereoselective nature of glucuronidation processes in human liver microsomes, indicating that different enantiomers of carvedilol may produce varying levels of glucuronidation products, potentially influencing their biological activities .
Q & A
Basic Research Questions
Q. How is Carvedilol beta-D-Glucuronide synthesized and characterized in preclinical studies?
Carvedilol beta-D-Glucuronide is typically synthesized via enzymatic glucuronidation using liver microsomes or recombinant UDP-glucuronosyltransferase (UGT) isoforms. Characterization involves liquid chromatography-tandem mass spectrometry (LC-MS/MS) to confirm the glucuronide conjugate and distinguish diastereomers. Nuclear magnetic resonance (NMR) is used to resolve stereochemical ambiguities, particularly for distinguishing β-D-glucuronide isomers . Reference standards (CAS 114869-83-9) are critical for method validation .
Q. What analytical methods are recommended for quantifying Carvedilol beta-D-Glucuronide in biological matrices?
High-performance liquid chromatography (HPLC) with UV or fluorescence detection is commonly employed, but LC-MS/MS offers higher sensitivity and specificity for low-concentration metabolites. Method validation must include assessments of linearity, precision, accuracy, and matrix effects, as outlined in pharmacopeial guidelines . For diastereomer separation, chiral stationary phases (e.g., cellulose- or amylose-based columns) are essential .
Q. Why is studying the glucuronide metabolite of Carvedilol pharmacologically significant?
Glucuronidation is a major Phase II metabolic pathway affecting Carvedilol's clearance and bioavailability. The beta-D-glucuronide conjugate may exhibit altered pharmacokinetics, renal excretion patterns, or potential drug-drug interactions (e.g., UGT inhibition by co-administered drugs). Understanding its stability and bioactivity is critical for interpreting clinical outcomes from Carvedilol therapy .
Advanced Research Questions
Q. How do experimental conditions influence diastereomer ratios in Carvedilol beta-D-Glucuronide synthesis?
Diastereomer ratios depend on enzymatic selectivity (e.g., UGT isoform specificity) and reaction pH. For instance, BcII-mediated hydrolysis of similar glucuronides showed a 7:3 diastereomer ratio due to stereoselective protonation at the active site . Analytical conditions (e.g., mobile phase composition in HPLC) can also artifactually alter observed ratios, necessitating rigorous method optimization .
Q. How can population pharmacokinetic (PK) modeling address variability in Carvedilol beta-D-Glucuronide exposure?
Population PK analyses integrate covariates like UGT polymorphisms, renal function, and age to explain inter-individual variability. Bayesian approaches are used to estimate PK parameters from sparse data, enabling dose adjustments in subpopulations (e.g., renal impairment). Validated LC-MS/MS methods are prerequisite for generating reliable concentration-time profiles .
Q. What computational tools predict the solubility and stability of Carvedilol beta-D-Glucuronide?
Quantitative structure-property relationship (QSPR) models, such as support vector regression (SVR), correlate molecular descriptors with solubility in supercritical CO₂ (scCO₂). For example, SVR-QSPR achieved a mean absolute relative deviation (MAARD) of 0.78 for Carvedilol solubility predictions . Molecular dynamics simulations further assess stability under physiological pH and temperature conditions .
Q. How to resolve contradictions in reported diastereomer bioactivity or metabolic fate?
Discrepancies often arise from differences in in vitro vs. in vivo systems (e.g., microsomal vs. hepatocyte models) or analytical methodologies. Cross-study comparisons require normalization to internal standards and validation using harmonized protocols (e.g., FDA Bioanalytical Method Validation). Meta-analyses of UGT isoform-specific activity data can clarify metabolic pathways .
Q. Methodological Considerations
Designing in vitro experiments to study Carvedilol beta-D-Glucuronide formation:
- Use human liver microsomes (HLM) or recombinant UGTs (e.g., UGT1A1, UGT2B7) with co-factors (UDPGA).
- Include controls for non-enzymatic degradation and matrix interferences.
- Optimize incubation time and protein concentration to avoid substrate depletion .
Validating chiral separation methods for diastereomers:
- Test multiple chiral columns (e.g., Chiralpak IA, IB) under varying mobile phase conditions (acetonitrile:buffer ratios).
- Assess resolution (Rs > 1.5), peak symmetry, and reproducibility across batches.
- Use synthesized pure diastereomers as reference materials .
Addressing instability of glucuronide metabolites in biological samples:
Properties
Molecular Formula |
C30H34N2O10 |
---|---|
Molecular Weight |
582.6 g/mol |
IUPAC Name |
(3S,4S,5S,6R)-6-[1-(9H-carbazol-4-yloxy)-3-[2-(2-methoxyphenoxy)ethylamino]propan-2-yl]oxy-3,4,5-trihydroxyoxane-2-carboxylic acid |
InChI |
InChI=1S/C30H34N2O10/c1-38-21-10-4-5-11-22(21)39-14-13-31-15-17(41-30-27(35)25(33)26(34)28(42-30)29(36)37)16-40-23-12-6-9-20-24(23)18-7-2-3-8-19(18)32-20/h2-12,17,25-28,30-35H,13-16H2,1H3,(H,36,37)/t17?,25-,26-,27-,28?,30+/m0/s1 |
InChI Key |
PUVQFGCELBOSRN-HEJFJQCXSA-N |
Isomeric SMILES |
COC1=CC=CC=C1OCCNCC(COC2=CC=CC3=C2C4=CC=CC=C4N3)O[C@H]5[C@H]([C@H]([C@@H](C(O5)C(=O)O)O)O)O |
Canonical SMILES |
COC1=CC=CC=C1OCCNCC(COC2=CC=CC3=C2C4=CC=CC=C4N3)OC5C(C(C(C(O5)C(=O)O)O)O)O |
Origin of Product |
United States |
Disclaimer and Information on In-Vitro Research Products
Please be aware that all articles and product information presented on BenchChem are intended solely for informational purposes. The products available for purchase on BenchChem are specifically designed for in-vitro studies, which are conducted outside of living organisms. In-vitro studies, derived from the Latin term "in glass," involve experiments performed in controlled laboratory settings using cells or tissues. It is important to note that these products are not categorized as medicines or drugs, and they have not received approval from the FDA for the prevention, treatment, or cure of any medical condition, ailment, or disease. We must emphasize that any form of bodily introduction of these products into humans or animals is strictly prohibited by law. It is essential to adhere to these guidelines to ensure compliance with legal and ethical standards in research and experimentation.