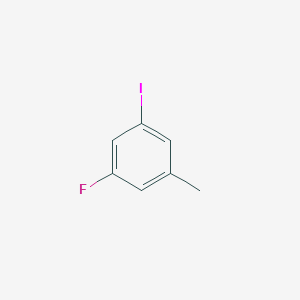
3-Fluoro-5-iodotoluene
Overview
Description
3-Fluoro-5-iodotoluene (CAS: 491862-84-1) is a halogenated aromatic compound with the molecular formula C₇H₆FI and a molecular weight of 236.03 g/mol. Structurally, it consists of a toluene backbone (methyl-substituted benzene) with fluorine and iodine substituents at the 3- and 5-positions, respectively. This compound is primarily utilized as an intermediate in organic synthesis, particularly in cross-coupling reactions (e.g., Suzuki-Miyaura) due to the reactivity of its iodine substituent .
Key properties include:
Preparation Methods
Copper(I)-Catalyzed Coupling Method
One documented method involves the copper(I) iodide-catalyzed coupling of 3-bromo-1H-1,2,4-triazole with 1-fluoro-3-iodo-5-methylbenzene in the presence of cesium carbonate base in dimethyl sulfoxide (DMSO) solvent under inert atmosphere at 100 °C for 20 hours. This reaction yields a product with 26.6% isolated yield after purification by silica gel chromatography.
Parameter | Details |
---|---|
Catalyst | Copper(I) iodide (CuI) |
Base | Cesium carbonate (Cs2CO3) |
Solvent | Dimethyl sulfoxide (DMSO) |
Temperature | 100 °C |
Reaction Time | 20 hours |
Atmosphere | Inert (Nitrogen) |
Yield | 26.6% isolated yield |
Purification | Silica gel column chromatography |
This method is suitable for coupling reactions but yields are moderate, and the reaction time is relatively long.
Multi-Step Synthesis via 3-Fluoro-5-iodoaniline Intermediate
A more industrially viable method involves the synthesis of 3-fluoro-5-iodoaniline as an intermediate, which can be further converted to 3-fluoro-5-iodotoluene derivatives. The process includes:
- Step 1: Ammonolysis of 3,4-difluoronitrobenzene in dimethyl sulfoxide at 80–100 °C for 1–5 hours to yield 3-fluoro-4-nitroaniline derivatives with yields up to 100%.
- Step 2: Iodination using iodine chloride in 50% ethanol-water solution at 30–50 °C for 2–6 hours.
- Step 3: Diazotization with sodium nitrite in concentrated sulfuric acid at 20–40 °C for 1–3 hours, followed by decomposition of the diazonium salt using sodium hypophosphite (Mordimehter reaction).
- Step 4: Reduction of the resulting compound with iron powder in acetic acid at 70–90 °C for 6–10 hours to yield 3-fluoro-5-iodoaniline with overall yields around 60–90%.
This method avoids expensive starting materials and complicated purification steps, making it suitable for industrial scale-up.
Step | Reaction Conditions | Yield (%) | Notes |
---|---|---|---|
1 | 3,4-Difluoronitrobenzene + NH3, DMSO, 80-100 °C, 1-5 h | 98-100 | Ammonolysis to compound III |
2 | Compound III + ICl, 50% EtOH/H2O, 30-50 °C, 2-6 h | Not specified | Iodination to compound IV |
3 | Compound IV + NaNO2, H2SO4 (98%), 20-40 °C, 1-3 h | Not specified | Diazotization and decomposition |
4 | Compound V + Fe powder, AcOH, 70-90 °C, 6-10 h | 88-90 | Reduction to 3-fluoro-5-iodoaniline |
This route is well-documented in patent CN117902987A and provides a total yield of approximately 61.9% with high purity (>99.8%) confirmed by 1H-NMR and GC analysis.
Method | Starting Material | Catalyst/Base | Solvent | Temperature | Reaction Time | Yield (%) | Purification | Industrial Suitability |
---|---|---|---|---|---|---|---|---|
Copper(I)-catalyzed coupling | 1-fluoro-3-iodo-5-methylbenzene | CuI, Cs2CO3 | DMSO | 100 °C | 20 h | 26.6 | Silica gel chromatography | Moderate |
Multi-step ammonolysis/iodination | 3,4-difluoronitrobenzene | NH3, ICl, NaNO2, Fe | DMSO, EtOH/H2O, AcOH | 20-100 °C | 1-10 h per step | ~62 total | Simple filtration, no column | High |
The multi-step method offers higher overall yield, lower cost starting materials, and simpler purification, making it more suitable for scale-up and industrial production.
- The ammonolysis step is critical and benefits from controlled temperature (80–100 °C) and reaction time (1–5 h) to maximize yield.
- Iodination with iodine chloride in aqueous ethanol is efficient and mild, avoiding harsh conditions.
- Diazotization and subsequent decomposition via the Mordimehter reaction under sodium hypophosphite conditions provide a safer alternative to fluorine pyrolysis, reducing hazards.
- Reduction with iron powder in acetic acid is effective for converting intermediates to the target amine.
- The copper-catalyzed coupling method, while useful for certain derivatives, suffers from lower yield and longer reaction times.
- Purity of the final product from the multi-step method exceeds 99.8%, confirmed by NMR and GC, indicating high-quality synthesis suitable for pharmaceutical applications.
The preparation of this compound is best achieved via a multi-step synthetic route involving ammonolysis of 3,4-difluoronitrobenzene, iodination, diazotization, and reduction steps. This method offers high yield, cost-effectiveness, and industrial scalability with straightforward purification. Copper(I)-catalyzed coupling methods provide alternative routes but with lower yields and longer reaction times. The detailed reaction conditions and yields provided here serve as a comprehensive guide for researchers and industrial chemists aiming to synthesize this compound efficiently.
Chemical Reactions Analysis
Types of Reactions: 3-Fluoro-5-iodotoluene undergoes several types of chemical reactions, including:
Substitution Reactions: The iodine atom can be substituted with other functional groups using reagents like palladium catalysts.
Oxidation Reactions: The methyl group can be oxidized to form corresponding carboxylic acids or aldehydes.
Reduction Reactions: The iodine atom can be reduced to form 3-fluorotoluene.
Common Reagents and Conditions:
Palladium Catalysts: Used in substitution reactions to replace the iodine atom with other functional groups.
Oxidizing Agents: Such as potassium permanganate or chromium trioxide, used in oxidation reactions.
Reducing Agents: Such as sodium borohydride or lithium aluminum hydride, used in reduction reactions.
Major Products Formed:
Substitution Products: Various substituted toluenes depending on the functional group introduced.
Oxidation Products: Carboxylic acids or aldehydes.
Reduction Products: 3-Fluorotoluene.
Scientific Research Applications
Chemistry: 3-Fluoro-5-iodotoluene is used as a building block in organic synthesis. Its unique reactivity makes it valuable for creating complex molecules in pharmaceuticals and agrochemicals .
Biology: In biological research, this compound is used to study the effects of fluorinated compounds on biological systems. It serves as a precursor for synthesizing biologically active molecules.
Medicine: The compound is explored for its potential in drug development. Its fluorine and iodine atoms can enhance the pharmacokinetic properties of drug candidates .
Industry: this compound is used in the production of specialty chemicals and materials. Its unique properties make it suitable for applications in electronics and advanced materials .
Mechanism of Action
The mechanism by which 3-Fluoro-5-iodotoluene exerts its effects depends on its application. In organic synthesis, it acts as a versatile intermediate, participating in various reactions to form complex molecules. In biological systems, its fluorine and iodine atoms can interact with molecular targets, influencing biological pathways and enhancing the activity of drug candidates .
Comparison with Similar Compounds
Comparison with Structural Isomers
The reactivity and applications of fluoro-iodotoluene derivatives depend heavily on the positions of substituents. Below is a comparative analysis of 3-fluoro-5-iodotoluene and its isomers:
Table 1: Structural Isomers of Fluoro-Iodotoluene
Key Findings:
Substituent Positioning: this compound and 2-fluoro-5-iodotoluene share the same molecular formula but differ in fluorine placement. The meta (3-) and para (5-) positions in this compound may enhance steric accessibility for cross-coupling reactions compared to ortho-substituted isomers .
Hazard Profile :
- Only this compound is explicitly flagged with H301 (acute toxicity), while other isomers lack detailed hazard data, possibly due to lower production volumes .
Comparison with Substituted Analogs
Halogenated toluenes with additional functional groups or substituents exhibit distinct properties:
Table 2: Substituted Halogenated Toluenes
Key Findings:
Chlorine Substitution :
- 5-Chloro-3-fluoro-2-iodotoluene (CAS: 939990-13-3) has a higher molecular weight (270.47 g/mol) due to chlorine. Its hazard profile includes H302 (harmful if swallowed) and respiratory irritation (H335 ), reflecting increased toxicity compared to this compound .
Functional Group Variation :
- 5-Fluoro-3-iodobenzoic acid (CAS: 23294-74-4) introduces a carboxylic acid group, altering reactivity (e.g., participation in esterification or amidation). Its lower price (¥29.00/250mg) suggests simpler synthesis or higher commercial availability .
Commercial and Industrial Considerations
- Production Scale : this compound is produced at metric ton scales , underscoring its industrial importance in synthesizing agrochemicals, pharmaceuticals, and materials .
- Cost : At ¥446.00/5g, this compound is more affordable than positional isomers like 2-fluoro-4-iodotoluene (¥9,800.00/5g) but pricier than functionalized analogs like 5-fluoro-3-iodobenzoic acid .
Biological Activity
3-Fluoro-5-iodotoluene is an organic compound with the molecular formula C₇H₆FI, characterized by a toluene ring substituted with a fluorine atom at the meta position and an iodine atom at the para position. This unique structure imparts distinct electronic properties, making it a valuable compound in organic synthesis and medicinal chemistry. This article explores its biological activity, potential applications, and relevant research findings.
The presence of both fluorine and iodine in this compound enhances its reactivity and potential for various chemical transformations. It can participate in nucleophilic substitutions and coupling reactions, including:
- Suzuki-Miyaura coupling
- Sonogashira coupling
- Negishi coupling
These reactions facilitate the introduction of diverse functional groups onto the aromatic ring, leading to complex molecules with potential pharmaceutical applications.
Medicinal Chemistry Applications
While no drugs currently contain this compound in its exact form, it serves as a precursor for synthesizing bioactive compounds. Its halogen substituents can significantly influence biological interactions:
- Fluorine : Often enhances metabolic stability and bioavailability of drugs.
- Iodine : Useful in radiopharmaceuticals for diagnostic imaging due to its unique properties .
Case Studies
Comparative Analysis of Similar Compounds
To understand the uniqueness of this compound, we can compare it with structurally similar compounds based on their properties and potential applications:
Compound Name | Formula | Key Features |
---|---|---|
3-Iodotoluene | C₇H₇I | Lacks fluorine; more reactive due to iodine |
4-Fluorotoluene | C₇H₇F | Lacks iodine; different electronic properties |
2-Fluoro-5-iodotoluene | C₇H₆FI | Different substitution pattern; similar reactivity |
4-Iodotoluene | C₇H₇I | Similar structure but lacks fluorine |
The combination of both fluorine and iodine in this compound provides distinct electronic characteristics that influence its reactivity and potential applications, setting it apart from these similar compounds.
Q & A
Basic Research Questions
Q. What are the optimal synthetic routes for 3-Fluoro-5-iodotoluene, and how do reaction conditions influence yield and purity?
- Methodological Answer :
- Direct iodination : Electrophilic iodination of 3-fluoro-5-methylbenzene using iodine monochloride (ICl) in acetic acid at 80°C yields this compound. Monitor reaction progress via TLC (Rf ~0.5 in hexane:ethyl acetate 9:1) .
- Cross-coupling : Suzuki-Miyaura coupling of 3-fluoro-5-bromotoluene with iodoboronic acids using Pd(PPh₃)₄ as a catalyst. Optimize solvent (THF vs. DMF) and base (K₂CO₃ vs. Cs₂CO₃) to minimize side products .
- Purification : Recrystallization in ethanol or column chromatography (silica gel, hexane:DCM 3:1) improves purity (>98% by GC-MS).
Q. How do the electronic effects of fluorine and iodine substituents influence the reactivity of this compound in electrophilic substitution reactions?
- Methodological Answer :
- Meta-directing effects : Fluorine’s strong electron-withdrawing nature directs incoming electrophiles to the meta position relative to itself, while iodine’s weaker electron-withdrawing effect stabilizes adjacent positions. Validate via nitration (HNO₃/H₂SO₄) and bromination (Br₂/FeBr₃) experiments, analyzing regioselectivity using H NMR .
- Computational validation : Use DFT calculations (Gaussian 09, B3LYP/6-31G*) to map electrostatic potential surfaces and predict reactive sites .
Advanced Research Questions
Q. How can researchers resolve contradictions in spectroscopic data (e.g., NMR shifts) for this compound derivatives?
- Methodological Answer :
- Cross-validation : Compare C NMR and X-ray crystallography data to confirm structural assignments. For example, discrepancies in aromatic proton shifts may arise from solvent effects or dynamic exchange processes .
- Dynamic effects : Variable-temperature NMR (VT-NMR) can identify conformational changes influencing chemical shifts. For instance, restricted rotation in ortho-substituted derivatives may split peaks .
Q. What experimental designs are suitable for probing the mechanistic role of this compound in palladium-catalyzed cross-coupling reactions?
- Methodological Answer :
- Kinetic studies : Use stopped-flow UV-Vis spectroscopy to track reaction intermediates. Vary catalyst loading (0.5–5 mol% Pd) and measure rate constants .
- Isotopic labeling : Introduce C or F labels to trace bond formation/cleavage steps. Analyze via LC-MS/MS .
- Computational modeling : Employ QM/MM simulations (e.g., ORCA) to map transition states and identify rate-limiting steps .
Q. Data Contradiction Analysis
Q. How should researchers address inconsistencies in reported melting points or solubility data for this compound?
- Methodological Answer :
- Reproducibility checks : Replicate synthesis and purification protocols from conflicting studies. For example, melting points may vary due to polymorphic forms or residual solvents. Use DSC (Differential Scanning Calorimetry) to confirm thermal behavior .
- Environmental factors : Control humidity and temperature during measurements. Solubility in DMSO vs. ethanol can differ by 20% depending on water content .
Properties
IUPAC Name |
1-fluoro-3-iodo-5-methylbenzene | |
---|---|---|
Source | PubChem | |
URL | https://pubchem.ncbi.nlm.nih.gov | |
Description | Data deposited in or computed by PubChem | |
InChI |
InChI=1S/C7H6FI/c1-5-2-6(8)4-7(9)3-5/h2-4H,1H3 | |
Source | PubChem | |
URL | https://pubchem.ncbi.nlm.nih.gov | |
Description | Data deposited in or computed by PubChem | |
InChI Key |
OIYMMFUTIUURQT-UHFFFAOYSA-N | |
Source | PubChem | |
URL | https://pubchem.ncbi.nlm.nih.gov | |
Description | Data deposited in or computed by PubChem | |
Canonical SMILES |
CC1=CC(=CC(=C1)I)F | |
Source | PubChem | |
URL | https://pubchem.ncbi.nlm.nih.gov | |
Description | Data deposited in or computed by PubChem | |
Molecular Formula |
C7H6FI | |
Source | PubChem | |
URL | https://pubchem.ncbi.nlm.nih.gov | |
Description | Data deposited in or computed by PubChem | |
DSSTOX Substance ID |
DTXSID60475932 | |
Record name | 3-Fluoro-5-iodotoluene | |
Source | EPA DSSTox | |
URL | https://comptox.epa.gov/dashboard/DTXSID60475932 | |
Description | DSSTox provides a high quality public chemistry resource for supporting improved predictive toxicology. | |
Molecular Weight |
236.02 g/mol | |
Source | PubChem | |
URL | https://pubchem.ncbi.nlm.nih.gov | |
Description | Data deposited in or computed by PubChem | |
CAS No. |
491862-84-1 | |
Record name | 3-Fluoro-5-iodotoluene | |
Source | EPA DSSTox | |
URL | https://comptox.epa.gov/dashboard/DTXSID60475932 | |
Description | DSSTox provides a high quality public chemistry resource for supporting improved predictive toxicology. | |
Record name | 5-Fluoro-3-iodotoluene | |
Source | European Chemicals Agency (ECHA) | |
URL | https://echa.europa.eu/information-on-chemicals | |
Description | The European Chemicals Agency (ECHA) is an agency of the European Union which is the driving force among regulatory authorities in implementing the EU's groundbreaking chemicals legislation for the benefit of human health and the environment as well as for innovation and competitiveness. | |
Explanation | Use of the information, documents and data from the ECHA website is subject to the terms and conditions of this Legal Notice, and subject to other binding limitations provided for under applicable law, the information, documents and data made available on the ECHA website may be reproduced, distributed and/or used, totally or in part, for non-commercial purposes provided that ECHA is acknowledged as the source: "Source: European Chemicals Agency, http://echa.europa.eu/". Such acknowledgement must be included in each copy of the material. ECHA permits and encourages organisations and individuals to create links to the ECHA website under the following cumulative conditions: Links can only be made to webpages that provide a link to the Legal Notice page. | |
Retrosynthesis Analysis
AI-Powered Synthesis Planning: Our tool employs the Template_relevance Pistachio, Template_relevance Bkms_metabolic, Template_relevance Pistachio_ringbreaker, Template_relevance Reaxys, Template_relevance Reaxys_biocatalysis model, leveraging a vast database of chemical reactions to predict feasible synthetic routes.
One-Step Synthesis Focus: Specifically designed for one-step synthesis, it provides concise and direct routes for your target compounds, streamlining the synthesis process.
Accurate Predictions: Utilizing the extensive PISTACHIO, BKMS_METABOLIC, PISTACHIO_RINGBREAKER, REAXYS, REAXYS_BIOCATALYSIS database, our tool offers high-accuracy predictions, reflecting the latest in chemical research and data.
Strategy Settings
Precursor scoring | Relevance Heuristic |
---|---|
Min. plausibility | 0.01 |
Model | Template_relevance |
Template Set | Pistachio/Bkms_metabolic/Pistachio_ringbreaker/Reaxys/Reaxys_biocatalysis |
Top-N result to add to graph | 6 |
Feasible Synthetic Routes
Disclaimer and Information on In-Vitro Research Products
Please be aware that all articles and product information presented on BenchChem are intended solely for informational purposes. The products available for purchase on BenchChem are specifically designed for in-vitro studies, which are conducted outside of living organisms. In-vitro studies, derived from the Latin term "in glass," involve experiments performed in controlled laboratory settings using cells or tissues. It is important to note that these products are not categorized as medicines or drugs, and they have not received approval from the FDA for the prevention, treatment, or cure of any medical condition, ailment, or disease. We must emphasize that any form of bodily introduction of these products into humans or animals is strictly prohibited by law. It is essential to adhere to these guidelines to ensure compliance with legal and ethical standards in research and experimentation.