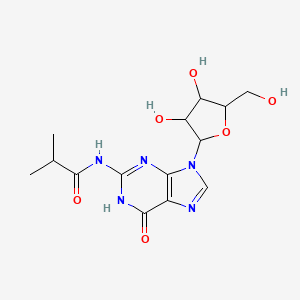
N2-Isobutyrylguanosine
- Click on QUICK INQUIRY to receive a quote from our team of experts.
- With the quality product at a COMPETITIVE price, you can focus more on your research.
Overview
Description
N2-Isobutyrylguanosine (CAS: 64350-24-9) is a chemically modified nucleoside where the exocyclic amino group of guanine is protected with an isobutyryl group. Its molecular formula is C₁₄H₁₉N₅O₆, with a molecular weight of 353.33 g/mol . This compound is widely employed as a precursor in oligonucleotide synthesis, particularly for introducing site-specific modifications in RNA and DNA. Its isobutyryl group enhances solubility and stability during chemical reactions while preventing unwanted side reactions at the N2 position .
Key applications include:
Preparation Methods
Synthetic Routes and Reaction Conditions: The synthesis of N-Isobutyrylguanosine typically involves the acylation of guanosine with isobutyryl chloride. The reaction is carried out in the presence of a base, such as pyridine, to facilitate the formation of the N-isobutyryl derivative. The general reaction scheme is as follows:
- Dissolve guanosine in an appropriate solvent, such as dimethylformamide (DMF).
- Add pyridine to the solution to act as a base.
- Slowly add isobutyryl chloride to the reaction mixture while maintaining a low temperature (0-5°C).
- Stir the reaction mixture for several hours at room temperature.
- Purify the product using column chromatography or recrystallization techniques.
Industrial Production Methods: Industrial production of N-Isobutyrylguanosine follows similar synthetic routes but on a larger scale. The process involves optimizing reaction conditions to maximize yield and purity. Key considerations include:
- Use of high-purity reagents and solvents.
- Efficient mixing and temperature control to ensure consistent reaction conditions.
- Implementation of purification steps, such as crystallization and filtration, to obtain the final product with high purity.
Chemical Reactions Analysis
Types of Reactions: N-Isobutyrylguanosine undergoes various chemical reactions, including:
Oxidation: The compound can be oxidized under specific conditions to form oxidized derivatives.
Reduction: Reduction reactions can convert N-Isobutyrylguanosine into reduced forms with different chemical properties.
Substitution: The isobutyryl group can be substituted with other functional groups through nucleophilic substitution reactions.
Common Reagents and Conditions:
Oxidation: Reagents such as potassium permanganate or hydrogen peroxide can be used under acidic or basic conditions.
Reduction: Reducing agents like sodium borohydride or lithium aluminum hydride are commonly employed.
Substitution: Nucleophiles such as amines or thiols can be used in the presence of a suitable catalyst.
Major Products Formed:
- Oxidized derivatives with altered electronic properties.
- Reduced forms with different functional groups.
- Substituted products with various functional groups replacing the isobutyryl group.
Scientific Research Applications
N-Isobutyrylguanosine has a wide range of applications in scientific research, including:
Chemistry: Used as a building block for the synthesis of oligoribonucleotides and other nucleic acid derivatives.
Biology: Employed in studies of RNA structure and function, as well as in the development of RNA-based therapeutics.
Medicine: Investigated for its potential use in antiviral and anticancer therapies due to its ability to interfere with nucleic acid metabolism.
Industry: Utilized in the production of nucleic acid-based materials and as a reagent in various chemical processes.
Mechanism of Action
The mechanism of action of N-Isobutyrylguanosine involves its incorporation into nucleic acids, where it can interfere with normal nucleic acid metabolism. The compound targets specific enzymes involved in nucleic acid synthesis and degradation, leading to the inhibition of viral replication or cancer cell proliferation. Key molecular targets include:
RNA polymerase: Inhibition of RNA polymerase activity, leading to reduced RNA synthesis.
Ribonuclease: Interference with ribonuclease activity, affecting RNA degradation pathways.
Comparison with Similar Compounds
The following table compares N2-Isobutyrylguanosine with structurally or functionally related nucleoside derivatives:
Key Research Findings:
Synthetic Efficiency: this compound demonstrates superior one-pot yields (81%) compared to traditional methods for 5′-azidonucleoside synthesis, outperforming uridine derivatives in scalability . In 2′-O-methylation reactions, however, it requires stringent conditions (e.g., SnCl₂ and diazomethane) to avoid undesired methylation at the nucleobase, yielding a mix of 2′-OMe and 3′-OMe regioisomers .
Functional Versatility: Unlike N6-Benzoyladenosine, which is primarily used in DNA synthesis, this compound is critical for RNA modifications, such as introducing photocleavable groups for time-resolved ribozyme studies . The 3′-O-TBDMS derivative shows unique compatibility with viral polymerases, a feature absent in unmodified guanosine .
Biological Activity
N2-Isobutyrylguanosine (N2-IBU-G) is a synthetic derivative of guanosine, characterized by the addition of an isobutyryl group at the nitrogen-2 position of the guanine base. Its molecular formula is C14H19N5O6, with a molecular weight of approximately 353.33 g/mol. This compound has garnered attention for its biological activity, particularly in the fields of cancer research and antiviral therapy.
This compound exhibits its biological effects primarily through the following mechanisms:
- Inhibition of Nucleic Acid Synthesis : The compound acts as a nucleoside analog, inhibiting RNA and DNA synthesis by interfering with the activity of key enzymes involved in nucleic acid metabolism, such as DNA polymerases and ribonucleotide reductases.
- Induction of Apoptosis : N2-IBU-G has been shown to induce apoptosis in cancer cells by activating caspases and other apoptotic pathways, leading to programmed cell death.
This compound demonstrates several important biochemical properties:
- Stability : The compound maintains stability under various storage conditions, with minimal degradation over time, making it suitable for long-term studies.
- Solubility : It is soluble in solvents such as dimethyl sulfoxide (DMSO) and methanol, facilitating its use in laboratory experiments.
Cellular Effects
In vitro studies have indicated that this compound effectively inhibits tumor growth and induces apoptosis in various cancer cell lines. Its cytotoxic effects are observed at specific concentrations without significant toxicity to normal cells .
Case Studies
- Antiviral Activity : this compound has been investigated for its antiviral potential. It is activated by thymidine kinase, which enhances its ability to inhibit viral replication processes. This characteristic makes it a candidate for antiviral therapies targeting various viruses.
- Cancer Research : In laboratory settings, N2-IBU-G has shown promise as an anticancer agent. Studies indicate that it can significantly reduce cell proliferation in cancerous tissues while exhibiting low cytotoxicity to healthy cells at effective doses .
Dosage Effects
Research indicates that the effects of this compound vary with dosage:
- Low Doses : At lower concentrations, it effectively inhibits tumor growth and induces apoptosis without notable toxicity.
- Higher Doses : Increased concentrations may lead to cytostatic effects, suppressing cell proliferation significantly .
Comparative Analysis
To understand the unique characteristics of this compound, it is beneficial to compare it with similar compounds:
Compound Name | Structure/Modification | Unique Features |
---|---|---|
Guanosine | Base nucleoside without modifications | Natural nucleoside; essential for RNA synthesis |
N6-Methyladenosine | Methyl group at nitrogen-6 position | Involved in RNA methylation processes |
5'-O-DMT-N2-Isobutyrylguanosine | Dimethoxytrityl protecting group | Used as a substrate for studying enzymatic reactions |
N-Acetylguanosine | Acetyl group at nitrogen-9 position | Enhances solubility and stability |
This compound's structural modification at the nitrogen-2 position enhances its biological activity compared to natural guanosine while retaining some structural characteristics that allow it to function similarly in biochemical pathways.
Q & A
Basic Research Questions
Q. What are the common synthetic routes for N2-Isobutyrylguanosine, and how can researchers optimize yield and purity?
this compound is typically synthesized through multi-step reactions involving protection/deprotection strategies. For example, a nine-step synthesis starting from guanosine involves N2-isobutyrylation followed by phosphoramidite derivatization for oligonucleotide incorporation . Key considerations include selecting protecting groups (e.g., o-nitrobenzyl for photocaging) and monitoring reaction intermediates via TLC or HPLC. Yield optimization may require adjusting stoichiometry, temperature, and catalyst use.
Q. Which analytical techniques are essential for characterizing this compound and its derivatives?
Standard methods include:
- NMR spectroscopy (¹H, ¹³C, and ³¹P for phosphorothiolate linkages).
- Mass spectrometry (MALDI-TOF or ESI-MS) to confirm molecular weight and purity.
- HPLC (reverse-phase or ion-pairing) for purity assessment . Ensure rigorous comparison with literature data for structural validation, especially when distinguishing between similar derivatives (e.g., methyl phosphate vs. phosphate dimers) .
Q. What safety protocols should be followed when handling this compound in the laboratory?
- Use personal protective equipment (PPE), including gloves and lab coats.
- Store the compound in a cool, dry environment (0–6°C for stability).
- Avoid inhalation or skin contact; work in a fume hood for solvent-based reactions .
Advanced Research Questions
Q. How can this compound be incorporated into oligonucleotides for mechanistic studies of RNA catalysis?
The phosphoramidite derivative of this compound enables solid-phase synthesis of RNA oligonucleotides with site-specific modifications. For example, 2′-O-photocaged 3′-S-thioguanosine derivatives are used to probe RNA cleavage mechanisms. Critical steps include optimizing coupling efficiency and deprotection conditions to preserve the phosphorothiolate linkage .
Q. What experimental strategies resolve contradictions in spectroscopic data for this compound derivatives?
Discrepancies in NMR or mass spectra may arise from:
- Tautomerism (e.g., keto-enol equilibria in guanine derivatives).
- Impurity co-elution in HPLC. Mitigate these by:
- Cross-validating with multiple techniques (e.g., 2D NMR or X-ray crystallography).
- Performing controlled degradation studies to identify labile functional groups .
Q. How should researchers design experiments to study the stability of this compound under varying pH and temperature conditions?
- Use accelerated stability testing (e.g., elevated temperatures or acidic/basic buffers) with HPLC monitoring.
- Quantify degradation products (e.g., deprotected guanosine) via calibration curves.
- Reference ICH guidelines for pharmaceutical stability protocols, adjusting parameters for research-scale studies .
Q. What methodologies are recommended for identifying gaps in existing literature on this compound applications?
- Conduct systematic reviews using databases like PubMed and SciFinder, filtering by keywords (e.g., "modified nucleosides," "RNA catalysis").
- Apply the PICO framework (Population, Intervention, Comparison, Outcome) to structure hypotheses. Example: "How does 3′-S-phosphorothiolate incorporation (Intervention) affect ribozyme cleavage rates (Outcome) compared to unmodified RNA (Comparison)?" .
Q. Methodological Guidance
Q. How can researchers formulate hypothesis-driven questions about this compound’s role in nucleotide analog development?
- Align with the FINER criteria : Ensure questions are Feasible, Interesting, Novel, Ethical, and Relevant.
- Example: "Does this compound enhance nuclease resistance in antisense oligonucleotides without compromising target binding affinity?" .
Q. What are best practices for documenting experimental procedures involving this compound in publications?
- Follow Beilstein Journal of Organic Chemistry guidelines :
- Detail synthetic steps (reagents, temperatures, reaction times).
- Provide characterization data (NMR shifts, HPLC retention times) for novel compounds.
- Deposit extensive datasets (e.g., crystallographic files) in supplementary materials .
Properties
Molecular Formula |
C14H19N5O6 |
---|---|
Molecular Weight |
353.33 g/mol |
IUPAC Name |
N-[9-[3,4-dihydroxy-5-(hydroxymethyl)oxolan-2-yl]-6-oxo-1H-purin-2-yl]-2-methylpropanamide |
InChI |
InChI=1S/C14H19N5O6/c1-5(2)11(23)17-14-16-10-7(12(24)18-14)15-4-19(10)13-9(22)8(21)6(3-20)25-13/h4-6,8-9,13,20-22H,3H2,1-2H3,(H2,16,17,18,23,24) |
InChI Key |
OXTYJSXVUGJSGM-UHFFFAOYSA-N |
Canonical SMILES |
CC(C)C(=O)NC1=NC2=C(C(=O)N1)N=CN2C3C(C(C(O3)CO)O)O |
Origin of Product |
United States |
Disclaimer and Information on In-Vitro Research Products
Please be aware that all articles and product information presented on BenchChem are intended solely for informational purposes. The products available for purchase on BenchChem are specifically designed for in-vitro studies, which are conducted outside of living organisms. In-vitro studies, derived from the Latin term "in glass," involve experiments performed in controlled laboratory settings using cells or tissues. It is important to note that these products are not categorized as medicines or drugs, and they have not received approval from the FDA for the prevention, treatment, or cure of any medical condition, ailment, or disease. We must emphasize that any form of bodily introduction of these products into humans or animals is strictly prohibited by law. It is essential to adhere to these guidelines to ensure compliance with legal and ethical standards in research and experimentation.