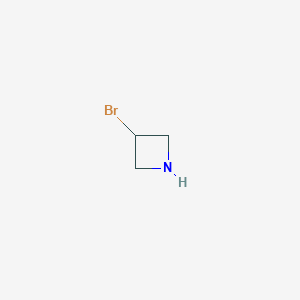
3-Bromoazetidine
Overview
Description
3-Bromoazetidine is a four-membered nitrogen-containing heterocycle with a bromine atom attached to the third carbon This compound is part of the azetidine family, known for their strained ring structures and significant reactivity
Mechanism of Action
Target of Action
3-Bromoazetidine is a small-membered azaheterocyclic compound . It is a versatile building block in the preparation of proteinogenic and non-proteinogenic amino acids and biologically active nitrogen-containing compounds . .
Mode of Action
The mode of action of this compound involves its reactivity as a strained compound, making it an excellent candidate for ring-opening and expansion reactions . It can undergo intramolecular nucleophilic displacement to yield a bicyclic aziridinium ion, which can then undergo alcoholysis .
Biochemical Pathways
This compound can be transformed into this compound-3-carboxylic acid derivatives via thermal isomerization . These derivatives contain a bromo-substituted carbon center, which is a useful moiety for functionalization . The functionalized azaheterocycles can undergo nucleophilic substitution with carbon, sulfur, oxygen, and nitrogen nucleophiles .
Result of Action
The result of this compound’s action is the production of a variety of functionally decorated heterocyclic compounds . These compounds are of interest from a biological point of view as well as for applications in the field of foldamers .
Action Environment
The action of this compound can be influenced by environmental factors such as temperature and solvent. For example, the refluxing of a precursor in a less nucleophilic solvent such as isopropanol can afford this compound as the sole product .
Biochemical Analysis
Biochemical Properties
3-Bromoazetidine plays a significant role in various biochemical reactions. It interacts with several enzymes and proteins, influencing their activity. For instance, this compound has been shown to interact with cytochrome P450 enzymes, which are crucial for the metabolism of many drugs and endogenous compounds. The interaction between this compound and these enzymes can lead to either inhibition or activation, depending on the specific enzyme and the context of the reaction . Additionally, this compound can form covalent bonds with nucleophilic amino acid residues in proteins, potentially altering their function .
Cellular Effects
This compound affects various types of cells and cellular processes. In cancer cells, it has been observed to inhibit cell proliferation by interfering with cell signaling pathways and inducing apoptosis . This compound can modulate gene expression by affecting transcription factors and other regulatory proteins. Moreover, this compound influences cellular metabolism by altering the activity of key metabolic enzymes, leading to changes in the levels of metabolites and energy production .
Molecular Mechanism
The molecular mechanism of this compound involves several key interactions at the molecular level. It can bind to the active sites of enzymes, either inhibiting or activating their catalytic activity. For example, this compound has been shown to inhibit the activity of certain kinases by binding to their ATP-binding sites . This inhibition can lead to downstream effects on cell signaling pathways and gene expression. Additionally, this compound can induce changes in gene expression by interacting with transcription factors and other DNA-binding proteins .
Temporal Effects in Laboratory Settings
In laboratory settings, the effects of this compound can change over time. This compound is relatively stable under standard laboratory conditions, but it can degrade over extended periods or under specific conditions such as high temperature or exposure to light . Long-term studies have shown that this compound can have sustained effects on cellular function, including prolonged inhibition of cell proliferation and induction of apoptosis in cancer cells . The exact temporal dynamics of these effects can vary depending on the experimental conditions and the specific cell types being studied.
Dosage Effects in Animal Models
The effects of this compound vary with different dosages in animal models. At low doses, it can have therapeutic effects, such as inhibiting tumor growth in cancer models . At higher doses, this compound can exhibit toxic effects, including hepatotoxicity and nephrotoxicity . These adverse effects are likely due to the compound’s interaction with critical enzymes and proteins in the liver and kidneys. Threshold effects have been observed, where a certain dosage level is required to achieve a therapeutic effect without causing significant toxicity .
Metabolic Pathways
This compound is involved in several metabolic pathways. It can be metabolized by cytochrome P450 enzymes, leading to the formation of various metabolites . These metabolites can further interact with other enzymes and cofactors, influencing metabolic flux and the levels of specific metabolites. For example, this compound can affect the glycolytic pathway by inhibiting key enzymes such as hexokinase and pyruvate kinase . This inhibition can lead to a decrease in glycolytic flux and a shift in cellular energy production.
Transport and Distribution
Within cells and tissues, this compound is transported and distributed through various mechanisms. It can be taken up by cells via passive diffusion or active transport mediated by specific transporters . Once inside the cell, this compound can bind to intracellular proteins and be distributed to different cellular compartments. The compound’s distribution can be influenced by its interactions with binding proteins and its physicochemical properties .
Subcellular Localization
The subcellular localization of this compound can affect its activity and function. This compound has been found to localize in the cytoplasm and the nucleus, where it can interact with various biomolecules . The localization of this compound can be directed by specific targeting signals or post-translational modifications that guide it to particular compartments or organelles. For instance, this compound can be targeted to the mitochondria, where it can influence mitochondrial function and energy production .
Preparation Methods
Synthetic Routes and Reaction Conditions: 3-Bromoazetidine can be synthesized through various methods. One common approach involves the amination, bromination, and base-induced cyclization of alkyl 2-(bromomethyl)acrylates. This method yields alkyl this compound-3-carboxylates, which can be further transformed into this compound derivatives via thermal isomerization .
Industrial Production Methods: In an industrial setting, the synthesis of this compound often involves the refluxing of specific intermediates in less nucleophilic solvents like isopropanol. This process ensures the selective formation of this compound as the sole product .
Chemical Reactions Analysis
Types of Reactions: 3-Bromoazetidine undergoes various chemical reactions, including nucleophilic substitution, ring-opening, and expansion reactions. These reactions are facilitated by the strained nature of the azetidine ring, making it highly reactive.
Common Reagents and Conditions:
Nucleophilic Substitution: Reagents such as potassium cyanide, potassium thiocyanate, sodium azide, and potassium phenoxide in solvents like dimethyl sulfoxide (DMSO) at elevated temperatures (e.g., 60°C) are commonly used.
Ring-Opening Reactions: These reactions often involve nucleophiles that attack the strained ring, leading to the formation of various functionalized products.
Major Products: The major products formed from these reactions include 3-cyano, 3-thiocyano, 3-azido, and 3-phenoxy azetidine derivatives .
Scientific Research Applications
3-Bromoazetidine has numerous applications in scientific research:
Chemistry: It serves as a valuable building block in the synthesis of complex molecules and heterocyclic compounds.
Biology: Its derivatives are used in the study of biological processes and as potential therapeutic agents.
Comparison with Similar Compounds
Aziridine: Another three-membered nitrogen-containing heterocycle, known for its high reactivity and use in organic synthesis.
Azetidine-2-carboxylic acid: A proline antagonist in plant tissue, used in the study of amino acid analogs.
Uniqueness: 3-Bromoazetidine is unique due to the presence of the bromine atom, which enhances its reactivity and potential for further functionalization. This makes it a valuable intermediate in the synthesis of various bioactive compounds and heterocyclic scaffolds .
Properties
IUPAC Name |
3-bromoazetidine | |
---|---|---|
Source | PubChem | |
URL | https://pubchem.ncbi.nlm.nih.gov | |
Description | Data deposited in or computed by PubChem | |
InChI |
InChI=1S/C3H6BrN/c4-3-1-5-2-3/h3,5H,1-2H2 | |
Source | PubChem | |
URL | https://pubchem.ncbi.nlm.nih.gov | |
Description | Data deposited in or computed by PubChem | |
InChI Key |
GJLIPAZOKHTKBR-UHFFFAOYSA-N | |
Source | PubChem | |
URL | https://pubchem.ncbi.nlm.nih.gov | |
Description | Data deposited in or computed by PubChem | |
Canonical SMILES |
C1C(CN1)Br | |
Source | PubChem | |
URL | https://pubchem.ncbi.nlm.nih.gov | |
Description | Data deposited in or computed by PubChem | |
Molecular Formula |
C3H6BrN | |
Source | PubChem | |
URL | https://pubchem.ncbi.nlm.nih.gov | |
Description | Data deposited in or computed by PubChem | |
DSSTOX Substance ID |
DTXSID20573766 | |
Record name | 3-Bromoazetidine | |
Source | EPA DSSTox | |
URL | https://comptox.epa.gov/dashboard/DTXSID20573766 | |
Description | DSSTox provides a high quality public chemistry resource for supporting improved predictive toxicology. | |
Molecular Weight |
135.99 g/mol | |
Source | PubChem | |
URL | https://pubchem.ncbi.nlm.nih.gov | |
Description | Data deposited in or computed by PubChem | |
CAS No. |
760159-34-0 | |
Record name | 3-Bromoazetidine | |
Source | EPA DSSTox | |
URL | https://comptox.epa.gov/dashboard/DTXSID20573766 | |
Description | DSSTox provides a high quality public chemistry resource for supporting improved predictive toxicology. | |
Retrosynthesis Analysis
AI-Powered Synthesis Planning: Our tool employs the Template_relevance Pistachio, Template_relevance Bkms_metabolic, Template_relevance Pistachio_ringbreaker, Template_relevance Reaxys, Template_relevance Reaxys_biocatalysis model, leveraging a vast database of chemical reactions to predict feasible synthetic routes.
One-Step Synthesis Focus: Specifically designed for one-step synthesis, it provides concise and direct routes for your target compounds, streamlining the synthesis process.
Accurate Predictions: Utilizing the extensive PISTACHIO, BKMS_METABOLIC, PISTACHIO_RINGBREAKER, REAXYS, REAXYS_BIOCATALYSIS database, our tool offers high-accuracy predictions, reflecting the latest in chemical research and data.
Strategy Settings
Precursor scoring | Relevance Heuristic |
---|---|
Min. plausibility | 0.01 |
Model | Template_relevance |
Template Set | Pistachio/Bkms_metabolic/Pistachio_ringbreaker/Reaxys/Reaxys_biocatalysis |
Top-N result to add to graph | 6 |
Feasible Synthetic Routes
Q1: What is the main application of 3-Bromoazetidine based on the research papers?
A1: The research papers primarily focus on using this compound as a building block for synthesizing more complex molecules, particularly new quinolone antibiotics [, ].
Q2: How is this compound incorporated into the structure of quinolone antibiotics?
A2: Researchers utilize this compound to introduce an azetidine ring at the C7 position of a quinolone nucleus []. This modification aims to enhance the antibacterial activity of the resulting quinolone derivatives.
Q3: Can you provide an example of a reaction pathway using this compound for synthesizing azetidine derivatives?
A3: One example involves reacting 1-azabicyclo[1.1.0]butane (ABB) with benzyl bromide to yield N-Benzyl-3-bromoazetidine []. This intermediate can then be further reacted with aliphatic amines to produce 3-aliphatic amino-substituted azetidine derivatives.
Q4: What is the significance of synthesizing new quinolone antibiotics using this compound?
A4: The emergence of antibiotic resistance, particularly methicillin-resistant Staphylococcus aureus (MRSA), poses a significant threat to global health. The research suggests that incorporating azetidine derivatives, synthesized using this compound, into the structure of quinolone antibiotics can potentially enhance their activity against MRSA compared to existing drugs like levofloxacin [].
Q5: Are there other applications of this compound besides antibiotic development?
A5: While the provided research focuses on antibiotic synthesis, this compound and similar azetidine derivatives have potential applications in various fields. These include the development of amino acid building blocks for peptide and protein modifications [, ].
Disclaimer and Information on In-Vitro Research Products
Please be aware that all articles and product information presented on BenchChem are intended solely for informational purposes. The products available for purchase on BenchChem are specifically designed for in-vitro studies, which are conducted outside of living organisms. In-vitro studies, derived from the Latin term "in glass," involve experiments performed in controlled laboratory settings using cells or tissues. It is important to note that these products are not categorized as medicines or drugs, and they have not received approval from the FDA for the prevention, treatment, or cure of any medical condition, ailment, or disease. We must emphasize that any form of bodily introduction of these products into humans or animals is strictly prohibited by law. It is essential to adhere to these guidelines to ensure compliance with legal and ethical standards in research and experimentation.