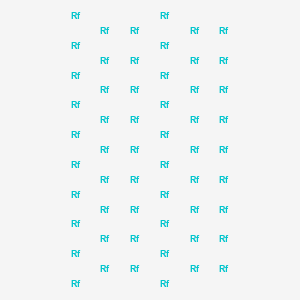
rutherfordium
- Click on QUICK INQUIRY to receive a quote from our team of experts.
- With the quality product at a COMPETITIVE price, you can focus more on your research.
Overview
Description
Rutherfordium (Rf), element 104, is a synthetic, radioactive transition metal in Group 4 of the periodic table. It was first synthesized in 1964 by bombarding californium-249 with carbon-12 nuclei . Its most stable isotope, ²⁶⁷Rf, has a half-life of ~48 minutes to 1.3 hours, making experimental studies challenging . Predicted to exhibit properties akin to its lighter homologs zirconium (Zr) and hafnium (Hf), Rf’s chemistry is influenced by relativistic effects due to its high atomic number, which alter electron configurations and bonding behavior .
Preparation Methods
Rutherfordium can be produced by the nuclear fusion of plutonium-242 with neon ions. The reaction is as follows: [ \text{^{242}Pu} + \text{^{22}Ne} \rightarrow \text{^{260}Rf} + 4 \text{n} ] Another method involves the bombardment of californium-249 with carbon ions: [ \text{^{249}Cf} + \text{^{12}C} \rightarrow \text{^{257}Rf} + 4 \text{n} ] These reactions are carried out in particle accelerators under highly controlled conditions .
Chemical Reactions Analysis
Gas-Phase Reactions
Rutherfordium forms volatile tetrahalides, a hallmark of group 4 chemistry. Key findings include:
Comparative Adsorption Enthalpies
Compound | Adsorption Enthalpy (ΔadsH, kJ/mol) |
---|---|
ZrCl₄ | −96 |
RfCl₄ | −102 |
HfCl₄ | −113 |
Data from gas chromatography experiments using SiO₂ columns .
Oxychloride Formation
RfCl₄ reacts with oxygen at elevated temperatures to form RfOCl₂ :
RfCl4+O2→RfOCl2+Cl2
Aqueous-Phase Behavior
In acidic solutions, Rf⁴⁺ ions hydrolyze and form complexes, mirroring hafnium’s chemistry but with distinct relativistic influences.
Hydrolysis and Ion Behavior
Extraction Studies
-
Hydrochloric Acid : Rf⁴⁺ co-elutes with Hf⁴⁺ and Zr⁴⁺ on cation-exchange resins, confirming group 4 affinity .
-
Hydrofluoric Acid : Weaker fluoride complexation compared to Hf/Zr, likely due to larger ionic radius .
Halogen Reactions
This compound reacts with halogens to form tetrahalides, consistent with its +4 oxidation state.
Tetrabromide (RfBr₄)
-
Synthesized similarly to RfCl₄, with comparable volatility trends .
-
Hydrolyzes in water to form RfOBr₂ :
RfBr4+H2O→RfOBr2+2HBr
Oxide Formation
RfO₂ is a refractory oxide analogous to HfO₂, stable at high temperatures :
Rf+O2→RfO2
Comparative Reactivity Table
Reaction Type | This compound Behavior | Hafnium Analogue |
---|---|---|
Chlorination | Forms volatile RfCl₄ | Forms HfCl₄ |
Hydrolysis (4+ ion) | RfO²⁺ in acidic solutions | HfO²⁺ |
Fluoride Complexation | [RfF₆]²⁻ (predicted) | [HfF₇]³⁻, [HfF₈]⁴⁻ |
Oxide Stability | High-melting RfO₂ | High-melting HfO₂ |
Relativistic Effects
Relativistic contraction of the 7s orbital and expansion of the 6d orbital alter Rf’s chemistry:
Unresolved Questions
-
Lower Oxidation States : +3 and +2 states are theorized but unconfirmed .
-
Organometallic Chemistry : No experimental data exist for Rf-carbon bonds.
This compound’s chemistry remains a frontier in transactinide research, with future studies focusing on relativistic effects and novel complex synthesis.
Scientific Research Applications
Rutherfordium (Rf), element 104 on the periodic table, is a synthetic element whose applications are currently confined to scientific research due to its radioactivity and short half-life . this compound's role is vital for expanding our understanding of nuclear chemistry, atomic theory, and the behavior of superheavy elements .
Nuclear Chemistry and Atomic Theory
- Exploring the Limits of the Periodic Table Studies of this compound and other superheavy elements provide insights into the limits of the periodic table and the potential for creating more stable heavy nuclei .
- Understanding Decay Properties Research focuses on synthesizing new elements and studying their decay properties, which can inform technological innovations in nuclear energy and materials science . Studying how superheavy elements decay could reveal new fundamental particles or unknown aspects of nuclear reactions .
- Relativistic Effects on Orbital Electrons this compound is used to study the impact of relativity on orbital electrons. As the first of the superheavy elements, it helps determine how relativity affects periodicity in the transactinide series .
Particle Physics and Radiology
- Synthesis Techniques The techniques developed for synthesizing this compound are also pivotal in particle physics and radiology, enhancing methods used in medical imaging and cancer treatment .
- Particle Accelerator Experiments this compound is used in particle research involving particle accelerator experiments. Through nuclear reactions, application of heavy ion beams, and review of decay data from particle accelerators, this compound can be used to study other superheavy, synthetic elements and the properties of the transactinide element series .
Chemical Properties and Compound Formation
- Gas Phase Chemistry this compound forms a volatile molecular tetrachloride, which is tetrahedral in shape, behaving as a typical group 4 element . this compound(IV) chloride is more volatile than hafnium(IV) chloride because its bonds are more covalent .
- Aqueous Chemistry this compound readily forms a hydrated Rf4+ ion in strong acid solutions and complexes in hydrochloric, hydrobromic, or hydrofluoric acid solutions . It forms a hexachlororutherfordate complex in chloride solutions, similar to hafnium and zirconium .
Potential Applications
- Catalysis: this compound's nanoparticles could potentially be explored as catalysts, similar to other precious metal nanoparticles like platinum and palladium . Its unique electronic configuration and high oxidation states could lead to novel catalytic properties and enhanced reactivity in certain chemical processes .
- Targeted Alpha Therapy: this compound compounds could potentially be used in nuclear medicine for targeted alpha therapy. The alpha particles emitted during decay allow for localized radiation damage to cancerous cells, minimizing effects on surrounding healthy tissue .
Table of Potential Applications
Case Studies
Because of its instability and the difficulty in producing it, there are no well-documented case studies regarding the applications of this compound outside of fundamental research . Studies on this compound have primarily focused on understanding its chemical properties and its place in the periodic table . These studies often involve comparing this compound's behavior with its lighter homologs in group 4, such as zirconium and hafnium .
Study of this compound Chloride Volatility
Experiments have been conducted to measure the volatility of this compound chloride and compare it with that of zirconium chloride and hafnium chloride . These studies aim to understand the relativistic effects that influence the chemical properties of this compound . The volatility of this compound chloride was found to be nearly the same as zirconium chloride, which is a break from periodic trends and is presumably caused by some relativistic effect .
Aqueous Chemistry Studies
Mechanism of Action
As a synthetic element, Rutherfordium does not have a biological role or mechanism of action in living organisms. Its effects are primarily studied in the context of nuclear reactions and particle physics. The production and study of this compound involve understanding its decay pathways and the energy released during its radioactive decay .
Comparison with Similar Compounds
Comparison with Group 4 Homologs: Zirconium and Hafnium
Chemical Behavior in Aqueous Solutions
- Ion Exchange Chromatography : Early studies demonstrated that Rf, Zr, and Hf elute similarly from cation exchange columns using ammonium α-hydroxyisobutyrate, while trivalent actinides (e.g., curium) remain adsorbed .
- Anion Exchange in HCl : In 12 M HCl, Rf and Hf form anionic chloride complexes that adsorb onto anion exchange resins, unlike trivalent actinides . This confirms Rf’s tetravalent (+4) oxidation state, consistent with Group 4 trends.
Table 1: Hydrolysis Constants of Tetravalent Elements
Element | log K₁₁ | log K₁₂ | log K₁₃ | log K₁₄ |
---|---|---|---|---|
Zr | 0.3 | -1.7 | -5.1 | -9.7 |
Hf | -0.25 | -2.4 | -6.0 | -10.7 |
Th | -3.2 | -6.93 | -11.7 | -15.9 |
Rf’s hydrolysis behavior is predicted to lie between Zr and Hf, with stronger hydrolysis than Th .
Solvent Extraction Studies
- Tributyl Phosphate (TBP) Extraction : Rf, Zr, and Hf exhibit similar distribution coefficients in TBP/HCl systems. At 0.25 M TBP, maximum extraction efficiency for tetravalent ions is achieved, with Rf’s behavior closer to Hf than Zr .
- Tri-iso-octylamine (TIOA) Extraction : Rf shows ~90% extraction in TIOA/HCl, comparable to Hf, indicating analogous anionic complex formation (e.g., [RfCl₆]²⁻) .
Table 2: Distribution Coefficients (Kd) in TBP (25°C)
Element | 6 M HCl | 8 M HCl |
---|---|---|
Rf | 120 | 300 |
Hf | 150 | 350 |
Zr | 80 | 200 |
Rf’s Kd values align more closely with Hf than Zr, suggesting similar chloride complex stability .
Ionic Radius and Coordination Chemistry
- Ionic Radius : Rf⁴⁺ has an estimated ionic radius of ~0.78 Å (six-coordinate) and ~0.92 Å (eight-coordinate), slightly larger than Hf⁴⁺ (0.71 Å and 0.83 Å, respectively) . This increase is attributed to relativistic expansion of the 6d orbitals.
- Coordination Preferences : Like Hf, Rf favors octahedral and eight-coordinate complexes in solution, differing from Zr’s tendency toward lower coordination numbers under similar conditions .
Deviations from Expected Trends: Relativistic Effects
Relativistic effects significantly influence Rf’s electronic structure:
- Electron Configuration : Theoretical models initially predicted a 7p-orbital contribution (e.g., [Rn]5f¹⁴6d¹7s²7p¹), but experimental data support a 6d-dominated configuration ([Rn]5f¹⁴6d²7s²), similar to Hf .
- Sublimation Enthalpy : Rf’s predicted sublimation enthalpy (~360 kJ/mol) is lower than Hf (~630 kJ/mol), indicating weaker metallic bonding due to relativistic contraction of the 7s orbital .
Comparison with Other Transactinides
- Lawrencium (Lr) : Unlike Lr, which exhibits a stabilized 7p¹/₂ orbital, Rf maintains typical Group 4 behavior, highlighting the nuanced impact of relativistic effects across the transactinide series .
- Dubnium (Db, Group 5) : Db’s +5 oxidation state contrasts with Rf’s +4, underscoring the stability of Group 4 tetravalency even in heavy elements .
Key Challenges :
Short half-lives limit detailed spectroscopic analysis.
Relativistic effects complicate extrapolation from lighter homologs.
Contamination from decay products requires ultra-pure separation methods .
Q & A
Basic Research Questions
Q. How is rutherfordium synthesized, and what challenges arise in producing sufficient quantities for experiments?
this compound is synthesized via heavy-ion nuclear fusion, typically by bombarding plutonium-242 or curium-248 targets with neon-22 or calcium-48 ions. Challenges include its short half-life (up to 1.3 hours for ²⁶⁷Rf) and low production yields (nanogram scales), necessitating rapid separation techniques like gas-phase chromatography or solvent extraction .
Q. What are the primary physical and chemical properties of this compound, and how are they predicted?
Predicted properties include a solid state at STP, HCP crystal structure, density of 17 g/cm³, and oxidation states of +4/+3. These are extrapolated using relativistic quantum calculations and comparisons with lighter group 4 homologs (Ti, Zr, Hf). Experimental validation is limited due to its short half-life .
Q. Why is this compound’s half-life critical for experimental design?
The short half-life imposes strict time constraints on experiments. Researchers use automated separation systems (e.g., Realtime Acquisition Graphics System [RAGS]) and rapid detection methods (PiPS detectors) to capture decay chains and isotopic signatures .
Q. What oxidation states are observed in this compound, and how do they compare to group 4 elements?
+4 is the dominant oxidation state, consistent with group 4 trends. However, relativistic effects may stabilize +3 states, differing from zirconium and hafnium. Solvent extraction studies with TTA (thenoyltrifluoroacetone) confirm these trends .
Q. How do researchers validate this compound’s position in the periodic table?
Comparative studies with homologs (e.g., gas-phase volatility experiments using SiO₂/Ta columns) reveal Rf’s behavior aligns closer to hafnium than thorium, confirming its group 4 placement .
Advanced Research Questions
Q. What methodological approaches determine this compound’s ionic radius in solution chemistry?
Ionic radii are estimated using solvent extraction with organic ligands (e.g., TTA, TBP) and plotting log Kₑq vs. ionic radius (Figure 63 in ). For 6-coordinate Rf⁴⁺, the radius is ~89 pm, validated against MCDF calculations .
Q. How do gas-phase chromatography and solvent extraction techniques differ in studying this compound’s chemical behavior?
Gas-phase methods (e.g., isothermal chromatography) measure volatility and adsorption enthalpies, while solvent extraction (e.g., using HCl/TBP systems) quantifies distribution coefficients. The former is optimal for rapid studies; the latter provides detailed thermodynamic data .
Q. How are discrepancies in this compound’s hydrolysis constants addressed across studies?
Discrepancies arise from differing experimental conditions (e.g., HCl concentration, temperature). Researchers use error propagation models and cross-validate results with relativistic density functional theory (DFT) to reconcile data .
Q. What computational models predict this compound’s electronic structure and bonding behavior?
Relativistic quantum mechanical methods, such as Dirac-Fock calculations and MCDF (Multi-Configuration Dirac-Fock), account for spin-orbit coupling effects. These models predict bond lengths and stability constants for Rf complexes .
Q. How do researchers optimize detector systems for this compound’s short-lived isotopes?
Silicon-based PiPS detectors and γ-ray spectroscopy are paired with automated data acquisition (e.g., DataMaster software) to capture decay events in real time. Time-stamped data filtering reduces background noise .
Q. Methodological Frameworks
- Experimental Design: Use the FINER criteria (Feasible, Interesting, Novel, Ethical, Relevant) to frame questions, ensuring alignment with relativistic chemistry or nuclear physics goals .
- Data Analysis: Employ error-weighted regression for decay chain analysis and Bayesian statistics to handle low-count data .
- Comparative Studies: Apply PICO (Population, Intervention, Comparison, Outcome) to structure homolog comparisons, e.g., Rf vs. Hf in solvent extraction .
Properties
CAS No. |
9012-56-0 |
---|---|
Molecular Formula |
Rf56 |
Molecular Weight |
14958.8 g/mol |
IUPAC Name |
rutherfordium |
InChI |
InChI=1S/56Rf |
InChI Key |
FLRBPEQFBHHKIL-UHFFFAOYSA-N |
Canonical SMILES |
[Rf].[Rf].[Rf].[Rf].[Rf].[Rf].[Rf].[Rf].[Rf].[Rf].[Rf].[Rf].[Rf].[Rf].[Rf].[Rf].[Rf].[Rf].[Rf].[Rf].[Rf].[Rf].[Rf].[Rf].[Rf].[Rf].[Rf].[Rf].[Rf].[Rf].[Rf].[Rf].[Rf].[Rf].[Rf].[Rf].[Rf].[Rf].[Rf].[Rf].[Rf].[Rf].[Rf].[Rf].[Rf].[Rf].[Rf].[Rf].[Rf].[Rf].[Rf].[Rf].[Rf].[Rf].[Rf].[Rf] |
Origin of Product |
United States |
Disclaimer and Information on In-Vitro Research Products
Please be aware that all articles and product information presented on BenchChem are intended solely for informational purposes. The products available for purchase on BenchChem are specifically designed for in-vitro studies, which are conducted outside of living organisms. In-vitro studies, derived from the Latin term "in glass," involve experiments performed in controlled laboratory settings using cells or tissues. It is important to note that these products are not categorized as medicines or drugs, and they have not received approval from the FDA for the prevention, treatment, or cure of any medical condition, ailment, or disease. We must emphasize that any form of bodily introduction of these products into humans or animals is strictly prohibited by law. It is essential to adhere to these guidelines to ensure compliance with legal and ethical standards in research and experimentation.