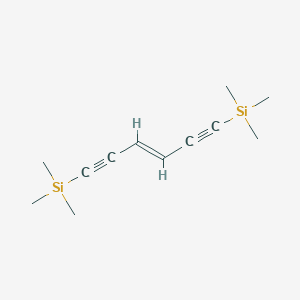
1,6-Bis-(trimethylsilyl)-3-hexen-1,5-diyne
- Click on QUICK INQUIRY to receive a quote from our team of experts.
- With the quality product at a COMPETITIVE price, you can focus more on your research.
Overview
Description
1,6-Bis-(trimethylsilyl)-3-hexen-1,5-diyne (CAS: N/A) is a conjugated enediyne compound featuring two terminal trimethylsilyl (TMS) groups and a central Z- or E-configured double bond. This compound is synthesized via coupling reactions, such as the coupling of TMS-acetylene with 1,2-dichloroethene under controlled conditions . The trimethylsilyl substituents confer steric bulk and electron-donating properties, influencing its reactivity and stability. Studies highlight its role as a precursor for annulenes (e.g., hexadehydro[18]annulene) and its unique reactivity with organoboranes, leading to structurally complex products .
Preparation Methods
Synthetic Routes and Reaction Conditions: 1,6-Bis-(trimethylsilyl)-3-hexen-1,5-diyne can be synthesized through a multi-step process involving the coupling of trimethylsilyl-protected alkynes. One common method involves the use of a palladium-catalyzed cross-coupling reaction between 1,6-dibromohexane and trimethylsilylacetylene in the presence of a base such as potassium carbonate. The reaction is typically carried out in an inert atmosphere to prevent oxidation and other side reactions.
Industrial Production Methods: While specific industrial production methods for this compound are not well-documented, the general approach involves scaling up the laboratory synthesis procedures. This includes optimizing reaction conditions, such as temperature, pressure, and solvent choice, to achieve higher yields and purity.
Chemical Reactions Analysis
Boration Reactions
1,6-Bis-(trimethylsilyl)-3-hexen-1,5-diyne undergoes 1,1-ethylboration and 1,2-allylboration with triethylborane and triallylborane, respectively. The reaction ratios depend on stereochemistry:
-
(E)-Isomer : Forms 1:1 ethylboration products.
-
(Z)-Isomer : Reacts only with one equivalent of triethylborane.
-
Triallylborane : Competes between 1,1-allylboration and 1,2-allylboration pathways .
Reagent | Reaction Type | Product Structure | Key Features |
---|---|---|---|
Triethylborane | 1,1-ethylboration | Organoboronated diynes | Stereoselective, 1:1 molar ratio |
Triallylborane | 1,1- or 1,2-allylboration | Allylboration products | Competitive pathways |
Hopf Cyclization
The compound undergoes 6π-electrocyclization on Au(111) surfaces, forming aromatic products like naphthalene and chrysene derivatives. Key findings:
-
Barrier Reduction : Reaction temperature is halved compared to bulk conditions (e.g., first cyclization completes below 160°C).
-
Mechanism : Involves gold-alkyne interactions that stabilize intermediates via density functional theory (DFT)-validated pathways .
-
Sequential Cyclizations :
Cross-Coupling Reactions
Participates in Pd/Cu-catalyzed alkynylation to form conjugated diynes:
-
Catalytic System : Pd(PPh₃)₂Cl₂ and CuI (3–5 mol%) in bases like diisopropylamine or triethylamine.
-
Applications : Synthesis of enediyne antibiotics and bioactive compounds .
-
Example : Coupling with trimethylsilylacetylene yields 1,3-diynyl products .
Catalyst | Conditions | Product Type | Yield Range |
---|---|---|---|
Pd/Cu (3–5 mol%) | Inert atmosphere, THF/DMF | 1,3-conjugated diynes | 79–94% |
Oxidation
Undergoes oxidation to form diketones or carboxylic acids, depending on reagents. The trimethylsilyl groups stabilize intermediates, influencing selectivity.
Electrophilic Addition
Reacts with electrophiles (e.g., acids, halogens) due to the electron-rich triple bonds. The silyl groups enhance stability, enabling controlled transformations.
Scientific Research Applications
Reactivity and Synthesis
1,6-Bis-(trimethylsilyl)-3-hexen-1,5-diyne exhibits notable reactivity in organic synthesis, particularly in reactions involving organoboron compounds. For instance, studies have demonstrated its ability to undergo 1,1-ethylboration with triethylborane and triallylborane, leading to complex product formations. The reactions can be monitored using NMR spectroscopy to analyze the resulting compounds' structures .
Key Reactions Involving this compound:
Reaction Type | Reagent | Product | Notes |
---|---|---|---|
1,1-Ethylboration | Triethylborane | Organoborane derivatives | Monitored by NMR spectroscopy |
1,2-Allylboration | Triallylborane | Mixed allylboration products | Complex reaction pathways observed |
Organoboration with 1-boraadamantane | 1-boraadamantane | Tetracyclic compounds | Rearrangement upon heating |
Applications in Organic Synthesis
The compound serves as a versatile building block in organic synthesis. Its ability to participate in cross-coupling reactions enhances its applicability in creating complex organic molecules. For example, it has been utilized in the Sonogashira reaction to form carbon-carbon bonds by coupling with terminal alkynes .
Case Studies
-
Sonogashira Coupling :
- Objective : To synthesize functionalized alkynes.
- Method : Coupling of this compound with various terminal alkynes using palladium catalysts.
- Outcomes : High yields of desired products were achieved, demonstrating the compound's effectiveness as a coupling partner.
- Reactivity with Boron Compounds :
Applications in Materials Science
The unique properties of this compound allow it to be used in the development of advanced materials. Its incorporation into polymer matrices can enhance thermal stability and mechanical strength.
Material Properties Table
Property | Description |
---|---|
Thermal Stability | High resistance to thermal degradation |
Mechanical Strength | Improved tensile properties |
Compatibility | Compatible with various polymer systems |
Mechanism of Action
The mechanism of action of 1,6-Bis-(trimethylsilyl)-3-hexen-1,5-diyne primarily involves its ability to undergo various chemical transformations due to the presence of reactive triple bonds and silyl groups. These transformations can lead to the formation of new chemical bonds and the introduction of functional groups into organic molecules. The molecular targets and pathways involved depend on the specific reactions and conditions used.
Comparison with Similar Compounds
Structural Analogs and Substituent Effects
The compound belongs to the 3-hexen-1,5-diyne family, where substituents at the 1,6-positions and double-bond configuration (Z/E) critically modulate chemical behavior. Key analogs include:
Substituent Impact :
- Electron-Donating Groups (TMS, MeO): Enhance stability and direct reactivity toward electrophilic additions (e.g., organoboration) .
- Aromatic Groups (Ph) : Promote π-π stacking, enabling AIE and supramolecular gel formation .
- Alkyl Chains (Pr): Increase hydrophobicity, favoring aggregation in nonpolar solvents .
Physical and Optical Properties
Biological Activity
1,6-Bis-(trimethylsilyl)-3-hexen-1,5-diyne is an organosilicon compound notable for its unique structure, which features two trimethylsilyl groups attached to a hexen-1,5-diyne backbone. This compound has garnered attention due to its potential biological activities, particularly in the field of cancer research. The molecular formula is C₁₂H₂₀Si₂ with a molecular weight of 220.46 g/mol. Its structure allows for various chemical reactions, making it a versatile building block in organic synthesis and materials science.
The compound's reactivity is primarily attributed to its alkyne groups, which can engage in multiple types of chemical reactions. The stability provided by the trimethylsilyl groups enhances its utility in synthetic applications.
Property | Value |
---|---|
Molecular Formula | C₁₂H₂₀Si₂ |
Molecular Weight | 220.46 g/mol |
Functional Groups | Alkynes, Trimethylsilyl |
Antitumor Activity
Recent studies have demonstrated that this compound exhibits significant antitumor properties. In a study involving the synthesis of various derivatives of this compound, it was found that several derivatives displayed potent cytotoxicity against KB (human oral cancer) and HeLa (cervical cancer) cell lines. The half-maximal inhibitory concentration (IC50) values for these compounds were reported to be below 10 μg/ml, indicating strong growth inhibition capabilities .
Case Study: Cytotoxicity Against Cancer Cells
A specific derivative synthesized from this compound was tested for its ability to inhibit cell growth in KB and HeLa cells:
Compound | Cell Line | IC50 (μg/ml) |
---|---|---|
Derivative A | KB | <10 |
Derivative B | HeLa | <10 |
Derivative C | KB | <10 |
Derivative D | HeLa | <10 |
This data suggests that modifications to the parent compound can enhance its biological activity.
The mechanism by which this compound exerts its antitumor effects involves cell cycle arrest and apoptosis induction. Specifically, one derivative was shown to arrest cancer cells in the G2/M phase and activate apoptotic pathways via Caspase-3 activation. Additionally, it caused microtubule depolymerization at low concentrations, which is critical for disrupting mitotic processes in cancer cells .
Synthesis and Variations
The synthesis of this compound can be achieved through palladium-catalyzed coupling reactions with aryl iodides. This method not only yields the desired compound but also allows for further modifications that can enhance biological activity. Variants of this compound have been synthesized to explore their biological properties further.
Q & A
Basic Research Questions
Q. What are the optimal synthetic routes for 1,6-Bis-(trimethylsilyl)-3-hexen-1,5-diyne, considering its sensitivity to moisture and oxygen?
- Methodological Answer : Synthesis should prioritize inert conditions (e.g., Schlenk line, argon glovebox) to prevent hydrolysis or oxidation of trimethylsilyl groups. Catalytic strategies (e.g., Sonogashira coupling for alkyne formation) should be evaluated for yield optimization. Post-synthesis purification via column chromatography under nitrogen can mitigate degradation . Theoretical frameworks, such as frontier molecular orbital analysis, may guide precursor selection to enhance reaction efficiency .
Q. How can spectroscopic techniques (NMR, IR, MS) be employed to characterize the structure and purity of this compound?
- Methodological Answer :
- ¹H/¹³C NMR : Focus on diagnostic signals for the ene-diyne backbone (e.g., alkene protons at δ 5–6 ppm, trimethylsilyl groups at δ 0–0.5 ppm).
- IR : Validate alkyne stretches (~2100–2260 cm⁻¹) and alkene C=C (~1600 cm⁻¹).
- MS : Confirm molecular ion peaks (e.g., ESI-MS for [M+H]⁺) and fragmentation patterns to assess purity.
- Cross-validate results with computational simulations (e.g., DFT for NMR chemical shifts) to resolve ambiguities .
Q. What safety protocols are critical when handling this compound?
- Methodological Answer :
- Storage : Use airtight containers under nitrogen at –20°C to prevent degradation. Avoid proximity to oxidizers or ignition sources due to potential flammability .
- Exposure Control : Employ fume hoods, nitrile gloves, and chemical-resistant goggles. Monitor degradation via periodic GC-MS analysis to detect byproducts like siloxanes .
Advanced Research Questions
Q. How can factorial design optimize reaction parameters (temperature, solvent, catalyst) for regioselective functionalization of the ene-diyne system?
- Methodological Answer : Implement a 2³ factorial design to test:
- Factors : Temperature (25°C vs. 60°C), solvent polarity (THF vs. DMF), catalyst loading (5 mol% vs. 10 mol%).
- Response Variables : Yield, regioselectivity ([2+2] vs. [4+2] cycloaddition).
- Analysis : Use ANOVA to identify significant interactions. For example, high polarity solvents may favor polar transition states, altering selectivity .
Q. What computational strategies (e.g., DFT, MD simulations) predict the compound’s reactivity in click chemistry or polymer applications?
- Methodological Answer :
- DFT : Calculate HOMO-LUMO gaps to predict susceptibility to electrophilic/nucleophilic attacks.
- MD Simulations : Model aggregation behavior in solvents to guide polymer design.
- AI Integration : Tools like COMSOL Multiphysics with machine learning can automate parameter optimization for large-scale synthesis .
Q. How can contradictions in reported reaction outcomes (e.g., competing cycloadditions) be resolved through mechanistic studies?
- Methodological Answer :
- In Situ Monitoring : Use real-time FT-IR or Raman spectroscopy to track intermediate formation.
- Isotopic Labeling : Introduce ¹³C at specific positions to elucidate bond-forming steps.
- Theoretical Alignment : Link experimental data to Woodward-Hoffmann rules or distortion/interaction models to rationalize selectivity .
Q. What advanced spectroscopic techniques (e.g., XAS, EPR) probe the electronic structure of the ene-diyne system under catalytic conditions?
- Methodological Answer :
- XAS (X-ray Absorption Spectroscopy) : Analyze edge features to study coordination changes during catalysis.
- EPR : Detect radical intermediates in photochemical reactions.
- Operando Studies : Combine spectroscopy with reactivity data to map catalytic cycles .
Q. Methodological Framework Integration
- Theoretical Linkage : Ground studies in concepts like molecular orbital theory or supramolecular chemistry to explain anomalous reactivity .
- Data Contradiction Analysis : Use triangulation (e.g., experimental, computational, and literature data) to validate hypotheses .
- Ethical Compliance : Follow CRDC guidelines (e.g., RDF2050108 for process control) to ensure reproducibility and safety .
Properties
Molecular Formula |
C12H20Si2 |
---|---|
Molecular Weight |
220.46 g/mol |
IUPAC Name |
trimethyl-[(E)-6-trimethylsilylhex-3-en-1,5-diynyl]silane |
InChI |
InChI=1S/C12H20Si2/c1-13(2,3)11-9-7-8-10-12-14(4,5)6/h7-8H,1-6H3/b8-7+ |
InChI Key |
AIXQKVZCTRWEGP-BQYQJAHWSA-N |
Isomeric SMILES |
C[Si](C)(C)C#C/C=C/C#C[Si](C)(C)C |
Canonical SMILES |
C[Si](C)(C)C#CC=CC#C[Si](C)(C)C |
Origin of Product |
United States |
Disclaimer and Information on In-Vitro Research Products
Please be aware that all articles and product information presented on BenchChem are intended solely for informational purposes. The products available for purchase on BenchChem are specifically designed for in-vitro studies, which are conducted outside of living organisms. In-vitro studies, derived from the Latin term "in glass," involve experiments performed in controlled laboratory settings using cells or tissues. It is important to note that these products are not categorized as medicines or drugs, and they have not received approval from the FDA for the prevention, treatment, or cure of any medical condition, ailment, or disease. We must emphasize that any form of bodily introduction of these products into humans or animals is strictly prohibited by law. It is essential to adhere to these guidelines to ensure compliance with legal and ethical standards in research and experimentation.