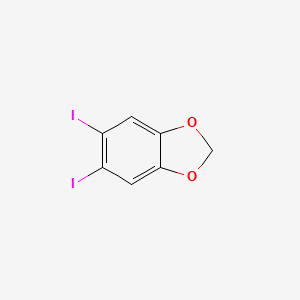
5,6-DIIODOBENZO(1,3)DIOXOLE
Overview
Description
5,6-Diiodobenzo[d][1,3]dioxole is a chemical compound with the molecular formula C7H4I2O2 and a molecular weight of 373.91 . It is used for research purposes .
Synthesis Analysis
Several methods have been reported for the synthesis of 5,6-Diiodobenzo[d][1,3]dioxole. One method involves the use of N-iodo-succinimide and trifluoroacetic acid in acetonitrile at 20℃ for 24 hours . Another method uses sulfuric acid, iodine, acetic acid, and periodic acid at 70℃ for 48 hours . Yet another method uses iodine and mercury (II) oxide in dichloromethane for 23 hours .Molecular Structure Analysis
The molecular structure of 5,6-Diiodobenzo[d][1,3]dioxole consists of a benzene ring fused with a 1,3-dioxole ring. The benzene ring is substituted at the 5 and 6 positions with iodine atoms .Scientific Research Applications
Antibacterial Applications
5,6-Diiodobenzo[d][1,3]dioxole derivatives demonstrate potential in antibacterial applications. A study highlighted that derivatives of a related compound, safrole (5-allylbenzo[d][1,3]dioxole), particularly safrole hydroperoxide, showed significant effectiveness against both Gram-positive and Gram-negative bacteria, suggesting potential medical applications (Khayyat & Al-Zahrani, 2014).
Antiepileptic Drug Synthesis
In the field of pharmaceuticals, 5,6-Diiodobenzo[d][1,3]dioxole analogs play a role in the synthesis of antiepileptic drugs. The stereoselective synthesis of the antiepileptic drug (R)-Stiripentol, involving the cross metathesis of 5-vinylbenzo[d][1,3]dioxole, highlights the compound's importance in drug development (El-Behairy & Sundby, 2013).
Medicinal Chemistry Research
In medicinal chemistry, 2,2-difluorobenzodioxole, a variant of 5,6-Diiodobenzo[d][1,3]dioxole, has been explored for creating metabolically stable derivatives of benzodioxole fragments. One such derivative, 4-chloro-2,2-difluoro[1,3]dioxole[4,5-c]pyridine, has been synthesized from simple materials, with potential for further functionalization in medicinal chemistry (Catalani, Paio, & Perugini, 2010).
Anticancer Research
Compounds related to 5,6-Diiodobenzo[d][1,3]dioxole have shown promise in anticancer research. A study synthesized and evaluated (Z)-2-(benzo[d][1,3]dioxol-5-yl) and related analogs against a panel of human cancer cell lines. Some analogs exhibited potent anticancer activity, suggesting the potential of these compounds in cancer treatment (Madadi et al., 2016).
Synthesis of Novel Compounds
5,6-Diiodobenzo[d][1,3]dioxole-related compounds are also involved in the synthesis of novel chemicals. One study reported the synthesis of various 2,2-dimethyl-6-(2-oxoalkyl)-1,3-dioxin-4-ones and their corresponding 6-substituted 4-hydroxy-2-pyrones. These syntheses provide pathways for creating new chemical entities with potential applications (Katritzky et al., 2005).
Safety and Hazards
Properties
IUPAC Name |
5,6-diiodo-1,3-benzodioxole | |
---|---|---|
Source | PubChem | |
URL | https://pubchem.ncbi.nlm.nih.gov | |
Description | Data deposited in or computed by PubChem | |
InChI |
InChI=1S/C7H4I2O2/c8-4-1-6-7(2-5(4)9)11-3-10-6/h1-2H,3H2 | |
Source | PubChem | |
URL | https://pubchem.ncbi.nlm.nih.gov | |
Description | Data deposited in or computed by PubChem | |
InChI Key |
BXUOIEPSOIEXIN-UHFFFAOYSA-N | |
Source | PubChem | |
URL | https://pubchem.ncbi.nlm.nih.gov | |
Description | Data deposited in or computed by PubChem | |
Canonical SMILES |
C1OC2=CC(=C(C=C2O1)I)I | |
Source | PubChem | |
URL | https://pubchem.ncbi.nlm.nih.gov | |
Description | Data deposited in or computed by PubChem | |
Molecular Formula |
C7H4I2O2 | |
Source | PubChem | |
URL | https://pubchem.ncbi.nlm.nih.gov | |
Description | Data deposited in or computed by PubChem | |
DSSTOX Substance ID |
DTXSID10569799 | |
Record name | 5,6-Diiodo-2H-1,3-benzodioxole | |
Source | EPA DSSTox | |
URL | https://comptox.epa.gov/dashboard/DTXSID10569799 | |
Description | DSSTox provides a high quality public chemistry resource for supporting improved predictive toxicology. | |
Molecular Weight |
373.91 g/mol | |
Source | PubChem | |
URL | https://pubchem.ncbi.nlm.nih.gov | |
Description | Data deposited in or computed by PubChem | |
CAS No. |
5876-52-8 | |
Record name | 5,6-Diiodo-2H-1,3-benzodioxole | |
Source | EPA DSSTox | |
URL | https://comptox.epa.gov/dashboard/DTXSID10569799 | |
Description | DSSTox provides a high quality public chemistry resource for supporting improved predictive toxicology. | |
Retrosynthesis Analysis
AI-Powered Synthesis Planning: Our tool employs the Template_relevance Pistachio, Template_relevance Bkms_metabolic, Template_relevance Pistachio_ringbreaker, Template_relevance Reaxys, Template_relevance Reaxys_biocatalysis model, leveraging a vast database of chemical reactions to predict feasible synthetic routes.
One-Step Synthesis Focus: Specifically designed for one-step synthesis, it provides concise and direct routes for your target compounds, streamlining the synthesis process.
Accurate Predictions: Utilizing the extensive PISTACHIO, BKMS_METABOLIC, PISTACHIO_RINGBREAKER, REAXYS, REAXYS_BIOCATALYSIS database, our tool offers high-accuracy predictions, reflecting the latest in chemical research and data.
Strategy Settings
Precursor scoring | Relevance Heuristic |
---|---|
Min. plausibility | 0.01 |
Model | Template_relevance |
Template Set | Pistachio/Bkms_metabolic/Pistachio_ringbreaker/Reaxys/Reaxys_biocatalysis |
Top-N result to add to graph | 6 |
Feasible Synthetic Routes
Disclaimer and Information on In-Vitro Research Products
Please be aware that all articles and product information presented on BenchChem are intended solely for informational purposes. The products available for purchase on BenchChem are specifically designed for in-vitro studies, which are conducted outside of living organisms. In-vitro studies, derived from the Latin term "in glass," involve experiments performed in controlled laboratory settings using cells or tissues. It is important to note that these products are not categorized as medicines or drugs, and they have not received approval from the FDA for the prevention, treatment, or cure of any medical condition, ailment, or disease. We must emphasize that any form of bodily introduction of these products into humans or animals is strictly prohibited by law. It is essential to adhere to these guidelines to ensure compliance with legal and ethical standards in research and experimentation.