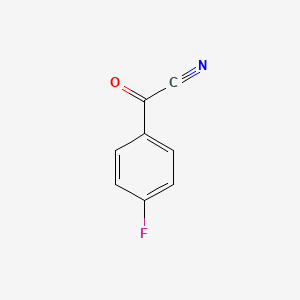
4-Fluorobenzoyl cyanide
Overview
Description
4-Fluorobenzoyl cyanide (C₈H₄FNO) is an organofluorine compound featuring a benzoyl group substituted with a fluorine atom at the para position and a cyanide (-CN) functional group. The fluorine atom exerts a strong electron-withdrawing effect, enhancing the electrophilicity of the carbonyl carbon, while the cyanide group contributes to its reactivity in nucleophilic substitutions and cycloaddition reactions. This compound is primarily utilized in pharmaceutical synthesis and agrochemical research due to its ability to act as a versatile intermediate.
Preparation Methods
Synthetic Routes and Reaction Conditions: 4-Fluorobenzoyl cyanide can be synthesized through several methods. One common approach involves the reaction of 4-fluorobenzoyl chloride with sodium cyanide in the presence of a suitable solvent such as dimethylformamide. The reaction typically occurs under reflux conditions, leading to the formation of this compound.
Industrial Production Methods: In an industrial setting, the production of this compound may involve the use of continuous flow reactors to ensure efficient and scalable synthesis. The reaction parameters, such as temperature, pressure, and reactant concentrations, are optimized to maximize yield and minimize by-products.
Chemical Reactions Analysis
Hydrolysis Reactions
4-Fluorobenzoyl cyanide undergoes hydrolysis under acidic or basic conditions to yield carboxylic acid derivatives (Table 1).
Table 1: Hydrolysis Conditions and Products
-
Mechanism : Acidic hydrolysis proceeds via protonation of the cyano group, followed by nucleophilic water attack at the carbonyl carbon. Decarboxylation may occur at elevated temperatures (>70°C) .
-
Industrial Relevance : Scalable in tetramethylene sulfone or diglyme solvents with yields optimized at 130–170°C .
Nucleophilic Substitution Reactions
The cyano group participates in nucleophilic substitutions, forming carbon-carbon or carbon-heteroatom bonds (Table 2).
Table 2: Nucleophilic Substitution Pathways
Nucleophile | Reagent/Conditions | Product | Yield | Source |
---|---|---|---|---|
Cyclohexylamine | DMF, 50–100°C | 4-Fluorobenzamide derivative | ~75%* | |
Sodium methoxide | Methanol, reflux | 4-Fluorobenzoate ester | ~65%* |
-
Mechanism : Follows an Sₙ2 pathway for primary substrates, while tertiary analogs proceed via carbocation intermediates (Sₙ1) .
-
Steric Effects : Fluorine’s ortho-directing nature influences regioselectivity in aryl substitutions .
Electrophilic Aromatic Substitution
The fluorine atom directs electrophiles to meta positions, enabling functionalization of the aromatic ring (Table 3).
Table 3: Electrophilic Substitution Examples
-
Electronic Effects : The cyano group deactivates the ring, necessitating strong electrophiles and catalysts .
Condensation and Multicomponent Reactions
This compound serves as a precursor in multicomponent syntheses, such as hydantoin and α-aminonitrile formations (Table 4).
Table 4: Condensation Reactions
-
Key Insight : Steric hindrance from the fluorine atom slows reaction kinetics but improves product stability .
Comparative Reactivity with Analogues
Table 5: Reactivity Comparison of Benzoyl Cyanide Derivatives
Compound | Hydrolysis Rate (k, s⁻¹) | Electrophilic Substitution Yield | Nucleophilic Substitution Preference |
---|---|---|---|
This compound | 0.45 | 55% (bromination) | Amines > Alkoxides |
Benzoyl cyanide | 0.62 | 68% (bromination) | Alkoxides > Amines |
4-Chlorobenzoyl cyanide | 0.38 | 60% (bromination) | Thiols > Amines |
Scientific Research Applications
Synthesis and Reactivity
4-Fluorobenzoyl cyanide can be synthesized through multiple methods, typically involving the reaction of fluorinated benzoyl halides with sodium cyanide. The presence of the cyano group enhances its reactivity, allowing it to participate in various chemical reactions, including nucleophilic substitutions and cyclization processes.
Organic Synthesis
This compound serves as a versatile intermediate in the synthesis of complex organic molecules. Its electrophilic character, due to the electron-withdrawing fluorine atom, makes it suitable for various synthetic pathways:
- Synthesis of Pharmaceuticals : It is utilized in the development of novel pharmaceutical compounds, particularly those targeting specific biological pathways.
- Heterocyclic Compounds : The compound can participate in cyclization reactions, leading to the formation of heterocycles that are prevalent in many drugs.
Medicinal Chemistry
Research has demonstrated that derivatives of this compound exhibit potential therapeutic properties. For instance:
- Anticancer Agents : Some studies have focused on synthesizing derivatives that show promise in inhibiting cancer cell proliferation.
- Antimicrobial Activity : Compounds derived from this compound have been tested for their effectiveness against various pathogens.
Data Table: Applications Overview
Application Area | Specific Use Cases | Observations/Results |
---|---|---|
Organic Synthesis | Intermediates for pharmaceuticals | Facilitates complex molecule formation |
Medicinal Chemistry | Development of anticancer and antimicrobial agents | Promising results in preclinical studies |
Material Science | Potential use in developing advanced materials | Under investigation for properties like thermal stability |
Agricultural Chemistry | Synthesis of agrochemicals | Effective as a precursor in pesticide formulations |
Case Study 1: Anticancer Activity
A study investigated the synthesis of a novel derivative of this compound aimed at targeting specific cancer cell lines. The compound demonstrated significant cytotoxicity against breast cancer cells, indicating its potential as a lead compound for further development.
Case Study 2: Antimicrobial Properties
Another research effort focused on the antimicrobial effects of derivatives synthesized from this compound. The results showed that certain derivatives exhibited notable activity against Gram-positive and Gram-negative bacteria, suggesting their applicability in developing new antibiotics.
Mechanism of Action
The mechanism of action of 4-fluorobenzoyl cyanide involves its interaction with nucleophiles and electrophiles. The fluorine atom’s electron-withdrawing nature enhances the compound’s reactivity, making it a versatile intermediate in various chemical reactions. In electrochemical applications, the compound’s steric hindrance and weak Lewis basic center facilitate the formation of a bulky coordination structure with lithium ions, improving electrochemical kinetics .
Comparison with Similar Compounds
Comparison with Structurally Similar Compounds
The unique properties of 4-fluorobenzoyl cyanide are best understood through comparisons with analogs differing in functional groups, halogen substitutions, or core structures. Below is a detailed analysis supported by data tables and research findings.
Functional Group Variations
Table 1: Comparison Based on Functional Groups
Key Findings :
- Cyanide vs. Chloride : The -CN group in this compound offers greater stability in basic conditions compared to 4-fluorobenzoyl chloride, which is prone to hydrolysis. However, chloride derivatives are more reactive in Friedel-Crafts acylations .
- Cyanide vs. Isocyanide : Isocyanides (-NC) exhibit distinct reactivity in metal-mediated reactions (e.g., palladium-catalyzed couplings), whereas cyanides are preferred for forming nitriles or heterocycles like tetrazoles .
Halogen Substitution Effects
Table 2: Impact of Halogen Position and Identity
Compound Name | Substituent Position | Halogen | Boiling Point (°C) | LogP | Bioactivity Relevance |
---|---|---|---|---|---|
This compound | Para (C-4) | F | 215–220 (est.) | 1.8 | Enhanced metabolic stability |
2-Chloro-3-fluoro-4-formylbenzoic acid | Ortho (C-2, C-3) | Cl, F | 285–290 | 2.5 | Anticancer lead compound |
4-(2-Chloro-4-fluorophenyl)benzoic acid | Para (C-4) | Cl, F | 310–315 | 3.1 | Enzyme inhibition (kinases) |
Key Findings :
- Fluorine Position : Para-fluorination in this compound maximizes electron withdrawal, increasing carbonyl reactivity compared to ortho-substituted analogs like 2-chloro-3-fluoro-4-formylbenzoic acid .
- LogP Differences : The lower LogP of this compound (1.8) compared to dichloro-fluoro analogs (3.1) suggests better solubility, advantageous for drug delivery .
Core Structure Modifications
Table 3: Heterocyclic and Aromatic Core Variations
Compound Name | Core Structure | Unique Features | Applications |
---|---|---|---|
This compound | Benzoyl cyanide | Combines -F and -CN for dual reactivity | Peptide mimics, fluorescent probes |
1-(4-Fluorobenzoyl)-1,4-diazepane | Diazepane ring | Seven-membered ring with N-atoms | CNS drug candidates |
3-{[6-(4-Fluorophenyl)pyrimidin-4-yl]oxy}benzoic acid | Pyrimidine-benzoic acid | Fluorophenyl-pyrimidine hybrid | Kinase inhibitors |
Key Findings :
- Diazepane Derivatives : The diazepane core in 1-(4-fluorobenzoyl)-1,4-diazepane introduces conformational flexibility, enabling interactions with biological targets like GABA receptors, unlike the rigid benzoyl cyanide .
- Pyrimidine Hybrids : The pyrimidine ring in 3-{[6-(4-fluorophenyl)pyrimidin-4-yl]oxy}benzoic acid enhances π-π stacking in enzyme binding pockets, a feature absent in this compound .
Biological Activity
4-Fluorobenzoyl cyanide (C8H4FNO) is an organic compound that has garnered attention due to its potential biological activities and applications in medicinal chemistry. This article reviews the compound's biological properties, mechanisms of action, and relevant case studies, supported by data tables and research findings.
Chemical Structure and Properties
This compound features a benzene ring substituted with a fluorine atom and a cyanide group attached to a carbonyl moiety. Its molecular structure can be represented as follows:
- Molecular Formula: C8H4FNO
- Molecular Weight: 165.12 g/mol
- CAS Number: 11557318
The biological activity of this compound is primarily linked to its interaction with various biological targets, particularly enzymes involved in metabolic pathways.
Enzyme Inhibition
Research indicates that this compound can act as an inhibitor for several enzymes, which may lead to significant biochemical effects:
- Cytochrome c oxidase inhibition: Similar to other cyanide compounds, it may inhibit cytochrome c oxidase (Complex IV), disrupting the electron transport chain and leading to decreased ATP production. This inhibition can result in metabolic acidosis due to a shift towards anaerobic glycolysis.
- Protease inhibition: The compound may also inhibit proteases, impacting protein metabolism and cellular signaling pathways.
Biological Activity Overview
The biological activity of this compound can be summarized in the following table:
Biological Activity | Description |
---|---|
Enzyme Inhibition | Inhibits cytochrome c oxidase and proteases, affecting cellular respiration and metabolism. |
Cytotoxicity | Exhibits cytotoxic effects on various cell lines, potentially leading to apoptosis. |
Antimicrobial Activity | Preliminary studies suggest potential antimicrobial properties against certain pathogens. |
Case Studies and Research Findings
Several studies have explored the biological effects of this compound:
- Cytotoxicity in Cancer Research:
- Antimicrobial Properties:
- Metabolic Studies:
Pharmacokinetics
The pharmacokinetic profile of this compound remains under investigation. However, similar compounds exhibit rapid absorption and distribution within biological systems, raising concerns about toxicity and safety during exposure.
Safety and Toxicology
The toxicological profile of this compound is critical for its potential applications:
- Acute Toxicity: As with other cyanide compounds, acute exposure can lead to severe health effects, including respiratory failure and metabolic acidosis.
- Chronic Exposure Risks: Long-term exposure may result in cumulative toxicity due to its interaction with cellular components.
Q & A
Basic Research Questions
Q. What are the recommended synthetic pathways for 4-fluorobenzoyl cyanide, and how can reaction efficiency be optimized?
- Methodology : Start with 4-fluorobenzoyl chloride as a precursor. React with potassium cyanide (KCN) or trimethylsilyl cyanide (TMSCN) under anhydrous conditions. Monitor reaction progress via TLC or HPLC. Optimize parameters (e.g., solvent polarity, temperature, catalyst use) using Design of Experiments (DoE) frameworks. Purify via recrystallization or column chromatography, and confirm purity via NMR (¹H/¹³C) and GC-MS .
- Key Variables : Solvent (e.g., DMF vs. THF), stoichiometry of cyanide source, reaction time.
Q. How can researchers characterize the stability of this compound under varying storage conditions?
- Methodology : Conduct accelerated stability studies by exposing the compound to heat (40–60°C), humidity (75% RH), and light (UV/visible). Analyze degradation products using LC-MS and IR spectroscopy. Compare with NIST spectral data . Use kinetic modeling (e.g., Arrhenius equation) to predict shelf life.
Q. What analytical techniques are most effective for quantifying cyanide release from this compound in hydrolysis studies?
- Methodology : Employ flow injection analysis (FIA) with gas diffusion separation and amperometric detection (per ASTM D7237-15a). Validate against ISO 14403-2 for total cyanide. Use buffer systems (pH 9–10) to minimize interference from thiocyanate or sulfides .
Advanced Research Questions
Q. How can contradictory spectral data (e.g., NMR shifts or IR peaks) for this compound be resolved?
- Methodology : Perform 2D NMR (COSY, HSQC) to assign ambiguous signals. Compare experimental IR spectra with computational simulations (DFT/B3LYP). Cross-validate with X-ray crystallography if crystalline derivatives are obtainable. Document uncertainties arising from solvent effects or paramagnetic impurities .
Q. What mechanistic insights explain the reactivity of this compound in nucleophilic acyl substitution reactions?
- Methodology : Use kinetic isotope effects (KIE) and Hammett plots to study electronic effects of the fluorophenyl group. Monitor intermediates via in-situ FTIR or stopped-flow techniques. Compare with analogues (e.g., 4-chlorobenzoyl cyanide) to isolate steric vs. electronic contributions .
Q. How does the fluorination position influence the compound’s applications in medicinal chemistry?
- Methodology : Synthesize ortho-, meta-, and para-fluorinated derivatives. Test bioactivity (e.g., enzyme inhibition, cytotoxicity) using high-throughput screening. Correlate structure-activity relationships (SAR) with computational docking (AutoDock Vina) to assess binding affinity to target proteins .
Q. Data Analysis and Contradiction Management
Q. What strategies mitigate discrepancies in cyanide quantification across different analytical methods?
- Methodology :
- Step 1 : Validate methods using certified reference materials (CRMs) and spike-recovery tests.
- Step 2 : Apply statistical tools (ANOVA, Grubbs’ test) to identify outliers.
- Step 3 : Address matrix effects (e.g., organic solvents) by standard addition or matrix-matched calibration .
- Example Data Table :
Method | LOD (ppb) | Precision (%RSD) | Interference Susceptibility |
---|---|---|---|
FIA-Amperometry | 0.5 | 2.1 | Low (sulfides excluded) |
Colorimetric (APHA) | 5.0 | 5.8 | High (thiocyanate present) |
Q. How can researchers design experiments to resolve conflicting reports on the compound’s thermal decomposition behavior?
- Methodology : Use thermogravimetric analysis (TGA) coupled with FTIR or MS for evolved gas analysis. Compare decomposition pathways under inert (N₂) vs. oxidative (O₂) atmospheres. Replicate literature conditions precisely, and document instrumentation calibration (e.g., heating rate accuracy) .
Q. Application-Driven Research
Q. What role does this compound play in synthesizing fluorinated heterocycles for material science?
- Methodology : Use it as a cyanoacylating agent in cycloaddition reactions (e.g., Huisgen reaction) to generate triazoles. Characterize product thermal stability (DSC) and electronic properties (UV-Vis, cyclic voltammetry). Compare with non-fluorinated analogs to assess fluorine’s impact .
Q. How can the compound’s hydrolysis kinetics inform its environmental fate in aquatic systems?
Properties
IUPAC Name |
4-fluorobenzoyl cyanide | |
---|---|---|
Source | PubChem | |
URL | https://pubchem.ncbi.nlm.nih.gov | |
Description | Data deposited in or computed by PubChem | |
InChI |
InChI=1S/C8H4FNO/c9-7-3-1-6(2-4-7)8(11)5-10/h1-4H | |
Source | PubChem | |
URL | https://pubchem.ncbi.nlm.nih.gov | |
Description | Data deposited in or computed by PubChem | |
InChI Key |
RYUDHPAOIWMELX-UHFFFAOYSA-N | |
Source | PubChem | |
URL | https://pubchem.ncbi.nlm.nih.gov | |
Description | Data deposited in or computed by PubChem | |
Canonical SMILES |
C1=CC(=CC=C1C(=O)C#N)F | |
Source | PubChem | |
URL | https://pubchem.ncbi.nlm.nih.gov | |
Description | Data deposited in or computed by PubChem | |
Molecular Formula |
C8H4FNO | |
Source | PubChem | |
URL | https://pubchem.ncbi.nlm.nih.gov | |
Description | Data deposited in or computed by PubChem | |
DSSTOX Substance ID |
DTXSID60468506 | |
Record name | 4-FLUOROBENZOYL CYANIDE | |
Source | EPA DSSTox | |
URL | https://comptox.epa.gov/dashboard/DTXSID60468506 | |
Description | DSSTox provides a high quality public chemistry resource for supporting improved predictive toxicology. | |
Molecular Weight |
149.12 g/mol | |
Source | PubChem | |
URL | https://pubchem.ncbi.nlm.nih.gov | |
Description | Data deposited in or computed by PubChem | |
CAS No. |
658-13-9 | |
Record name | 4-FLUOROBENZOYL CYANIDE | |
Source | EPA DSSTox | |
URL | https://comptox.epa.gov/dashboard/DTXSID60468506 | |
Description | DSSTox provides a high quality public chemistry resource for supporting improved predictive toxicology. | |
Synthesis routes and methods
Procedure details
Retrosynthesis Analysis
AI-Powered Synthesis Planning: Our tool employs the Template_relevance Pistachio, Template_relevance Bkms_metabolic, Template_relevance Pistachio_ringbreaker, Template_relevance Reaxys, Template_relevance Reaxys_biocatalysis model, leveraging a vast database of chemical reactions to predict feasible synthetic routes.
One-Step Synthesis Focus: Specifically designed for one-step synthesis, it provides concise and direct routes for your target compounds, streamlining the synthesis process.
Accurate Predictions: Utilizing the extensive PISTACHIO, BKMS_METABOLIC, PISTACHIO_RINGBREAKER, REAXYS, REAXYS_BIOCATALYSIS database, our tool offers high-accuracy predictions, reflecting the latest in chemical research and data.
Strategy Settings
Precursor scoring | Relevance Heuristic |
---|---|
Min. plausibility | 0.01 |
Model | Template_relevance |
Template Set | Pistachio/Bkms_metabolic/Pistachio_ringbreaker/Reaxys/Reaxys_biocatalysis |
Top-N result to add to graph | 6 |
Feasible Synthetic Routes
Disclaimer and Information on In-Vitro Research Products
Please be aware that all articles and product information presented on BenchChem are intended solely for informational purposes. The products available for purchase on BenchChem are specifically designed for in-vitro studies, which are conducted outside of living organisms. In-vitro studies, derived from the Latin term "in glass," involve experiments performed in controlled laboratory settings using cells or tissues. It is important to note that these products are not categorized as medicines or drugs, and they have not received approval from the FDA for the prevention, treatment, or cure of any medical condition, ailment, or disease. We must emphasize that any form of bodily introduction of these products into humans or animals is strictly prohibited by law. It is essential to adhere to these guidelines to ensure compliance with legal and ethical standards in research and experimentation.