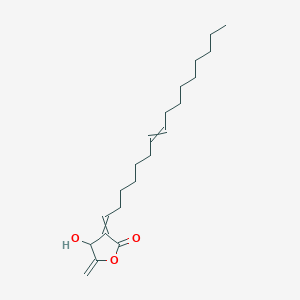
Isoobtusilactone B
- Click on QUICK INQUIRY to receive a quote from our team of experts.
- With the quality product at a COMPETITIVE price, you can focus more on your research.
Overview
Description
Isoobtusilactone B is a naturally occurring butanolide lactone first isolated from Lindera obtusiloba Blume, a plant traditionally used in East Asian medicine . Structurally, it belongs to the α-methylene-γ-butyrolactone family, characterized by a γ-lactone core with an exocyclic methylene group and a long endoolefinic alkyl side chain (–(CH₂)₅–CH=CH–(CH₂)₇–CH₃) . This compound has been misassigned in public databases (e.g., PubChem CID: 180554 lists an incorrect structure lacking the central C7′-C8′ double bond), with the correct structure corresponding to PubChem CID: 101286261 (C₂₁H₃₄O₃) .
Preparation Methods
Synthetic Routes and Reaction Conditions
The synthesis of isoobtusilactone B typically involves the use of starting materials such as cinnamic acid derivatives. The synthetic route often includes steps like esterification, cyclization, and oxidation. For instance, one common method involves the esterification of cinnamic acid followed by cyclization to form the lactone ring, and subsequent oxidation to yield this compound .
Industrial Production Methods
Industrial production of this compound is less common due to the complexity of its synthesis. advancements in biotechnological methods, such as the use of plant cell cultures, have shown promise in producing this compound on a larger scale .
Chemical Reactions Analysis
Structural Context of Butanolides
Butanolides are γ-lactones with a polyketide-derived backbone, often featuring conjugated double bonds and hydroxyl or alkyl substituents. For example:
-
Isoobtusilactone A : Contains an α-alkylidene-γ-lactone skeleton with a Δ⁴,⁵ unsaturation and a hydroxyl group at C-3 .
-
Isoobtusilactone B : While not explicitly described in the sources, structural analogs suggest potential reactivity at the lactone ring or conjugated side chains.
Reactivity of α-Alkylidene-γ-Lactones
The reactivity of isoobtusilactone A involves:
-
Electrophilic addition at the α,β-unsaturated lactone system.
-
Hydrolysis of the lactone ring under acidic or basic conditions, forming carboxylic acid derivatives.
-
Michael addition at the α,β-unsaturated carbonyl site, a common reaction for similar butanolides .
Biological Activity and Mechanistic Insights
While not a direct chemical reaction, isoobtusilactone A’s biological effects on Trypanosoma cruzi highlight its interaction with biomolecules:
Gaps in this compound Research
No peer-reviewed studies on this compound were identified in the provided sources. This compound may represent:
-
A structural isomer or homolog of isoobtusilactone A.
-
An understudied analog with uncharacterized reactivity.
Recommendations for Further Study
To characterize this compound’s reactions, the following approaches could be employed:
-
Synthetic modification of the lactone ring or side chains.
-
In vitro reactivity assays with nucleophiles (e.g., thiols, amines).
-
Computational modeling to predict sites of electrophilic or nucleophilic attack.
The absence of this compound in the literature underscores the need for targeted studies to elucidate its chemical behavior. Researchers should prioritize structural elucidation and comparative analyses with isoobtusilactone A.
Scientific Research Applications
Chemistry: It serves as a valuable intermediate in the synthesis of more complex molecules.
Biology: Isoobtusilactone B has shown significant activity against various biological targets, making it a subject of interest in biochemical studies.
Medicine: Its anticancer properties have been demonstrated in several studies, where it induces apoptosis in cancer cells.
Mechanism of Action
The mechanism by which isoobtusilactone B exerts its effects involves several molecular targets and pathways:
Apoptosis Induction: this compound induces apoptosis in cancer cells through the mitochondrial pathway, involving the release of cytochrome c and activation of caspases.
Reactive Oxygen Species (ROS) Generation: The compound increases the production of ROS, which leads to oxidative stress and cell death in cancer cells.
Inhibition of Enzymes: This compound inhibits various enzymes involved in inflammation and microbial growth.
Comparison with Similar Compounds
Structural and Functional Comparison with Similar Compounds
Butanolide lactones share a γ-lactone core but differ in side-chain configurations and biological activities. Below is a comparative analysis of Isoobtusilactone B and its analogs:
Isoobtusilactone A
- Structure : Similar γ-lactone core but distinct alkyl side-chain modifications.
- Biological Activities: Anticancer Effects: Induces apoptosis in hepatoma (Hep G2), breast (MCF-7, MDA-MB-231), and lung (A549) cancer cells via reactive oxygen species (ROS)-mediated pathways, mitochondrial depolarization, and caspase activation . Antiparasitic Activity: Exhibits potent activity against Trypanosoma cruzi (Chagas disease pathogen), inhibiting metacyclogenesis (43% inhibition at 2.75 μM) and host-cell infection by damaging mitochondrial ultrastructure . Synergism: Combines with majoranolide to reduce drug doses while enhancing efficacy against T. cruzi amastigotes .
Obtusilactone A
- Structure : Shares the α-methylene-γ-butyrolactone core but differs in alkyl chain length (C17 vs. C21 in this compound).
- Biological Activities :
Majoranolide
- Structure: A butanolide dimer with distinct side-chain topology.
- Biological Activities :
Comparative Data Table
Q & A
Basic Research Questions
Q. What experimental methodologies are commonly used to study the apoptotic mechanisms of isoobtusilactone B in cancer cells?
this compound induces apoptosis via mitochondrial pathways and reactive oxygen species (ROS) generation. Key methods include:
- Flow cytometry to quantify sub-G1 populations and cell cycle arrest (G2/M phase) .
- Western blotting to measure apoptosis-related proteins (e.g., Bax/Bcl-2 ratios, caspase-3/9 activation, PARP cleavage) .
- ROS detection assays (e.g., DCFH-DA probes) to validate ROS involvement, with controls using antioxidants like N-acetylcysteine (NAC) .
- Transwell assays to assess anti-migration and anti-invasion effects .
Q. How is the specificity of this compound’s anticancer activity validated across different cell lines?
Researchers employ:
- Dose-response curves (e.g., IC50 calculations) in multiple cancer cell lines (e.g., A549 lung cancer, HepG2 hepatoma) .
- Comparative studies with structurally related compounds (e.g., isoobtusilactone A, subamolides) to isolate structure-activity relationships .
- Normal cell controls (e.g., non-cancerous fibroblasts) to confirm selective toxicity .
Q. What molecular targets are implicated in this compound’s cell cycle disruption?
this compound upregulates cell cycle inhibitors (p21/WAF1, p27) and tumor suppressor p53, validated via:
- qPCR and immunoblotting to measure mRNA/protein levels .
- siRNA knockdown experiments to confirm functional roles of p53/p21 in G2/M arrest .
Advanced Research Questions
Q. How can researchers resolve contradictions in this compound’s ROS-dependent vs. ROS-independent apoptotic effects across studies?
Contradictions may arise from cell-type-specific ROS thresholds or experimental conditions. Methodological strategies include:
- ROS scavenger titration (e.g., varying NAC concentrations) to identify threshold effects .
- Time-course experiments to distinguish early ROS bursts from downstream apoptotic events .
- Multi-omics integration (transcriptomics/proteomics) to map ROS-independent pathways, such as direct BAF1 protein interactions .
Q. What in vivo experimental designs are optimal for evaluating this compound’s pharmacokinetics and toxicity?
Advanced models require:
- Pharmacokinetic profiling via HPLC-MS to track bioavailability and metabolite formation .
- Xenograft mouse models with tumor volume monitoring and histopathological analysis of organ toxicity (e.g., liver, kidneys) .
- Dose optimization using escalating doses to balance efficacy (tumor suppression) and safety (body weight/hematological parameters) .
Q. How can computational approaches enhance understanding of this compound’s interaction with barrier-to-autointegration factor 1 (BAF1)?
Molecular docking and dynamics simulations are used to:
- Predict binding affinities and key residues in BAF1-ligand interactions .
- Validate findings with surface plasmon resonance (SPR) or isothermal titration calorimetry (ITC) for empirical binding constants .
Q. What strategies address this compound’s potential chemoresistance mechanisms in cancer cells?
Resistance mechanisms (e.g., upregulated drug efflux pumps) can be investigated via:
- ABC transporter inhibition assays (e.g., verapamil for P-glycoprotein) to restore cytotoxicity .
- Combination therapy screens with standard chemotherapeutics (e.g., cisplatin) to identify synergistic effects .
Q. Methodological Considerations
- Data Interpretation : When comparing studies, note variations in this compound purity, solvent (DMSO vs. ethanol), and cell culture conditions (e.g., serum concentration) .
- Reproducibility : Follow standardized protocols for ROS assays (e.g., avoiding light exposure for fluorescent probes) and apoptosis markers (e.g., caspase activity normalization) .
- Ethical Reporting : Adhere to journal guidelines for supplemental data (e.g., raw flow cytometry files in Supplementary Materials) and avoid duplicative figure/table descriptions .
Properties
Molecular Formula |
C21H34O3 |
---|---|
Molecular Weight |
334.5 g/mol |
IUPAC Name |
3-hexadec-7-enylidene-4-hydroxy-5-methylideneoxolan-2-one |
InChI |
InChI=1S/C21H34O3/c1-3-4-5-6-7-8-9-10-11-12-13-14-15-16-17-19-20(22)18(2)24-21(19)23/h10-11,17,20,22H,2-9,12-16H2,1H3 |
InChI Key |
RWMHGSZPUZTJHR-UHFFFAOYSA-N |
Canonical SMILES |
CCCCCCCCC=CCCCCCC=C1C(C(=C)OC1=O)O |
Origin of Product |
United States |
Disclaimer and Information on In-Vitro Research Products
Please be aware that all articles and product information presented on BenchChem are intended solely for informational purposes. The products available for purchase on BenchChem are specifically designed for in-vitro studies, which are conducted outside of living organisms. In-vitro studies, derived from the Latin term "in glass," involve experiments performed in controlled laboratory settings using cells or tissues. It is important to note that these products are not categorized as medicines or drugs, and they have not received approval from the FDA for the prevention, treatment, or cure of any medical condition, ailment, or disease. We must emphasize that any form of bodily introduction of these products into humans or animals is strictly prohibited by law. It is essential to adhere to these guidelines to ensure compliance with legal and ethical standards in research and experimentation.