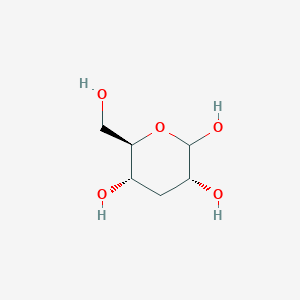
3-Deoxy-D-glucopyranose
- Click on QUICK INQUIRY to receive a quote from our team of experts.
- With the quality product at a COMPETITIVE price, you can focus more on your research.
Overview
Description
3-Deoxy-D-glucopyranose is a derivative of glucose where the hydroxyl group at the third carbon is replaced by a hydrogen atom. This modification results in a compound with the molecular formula C6H12O5. It is a significant molecule in carbohydrate chemistry and has various applications in scientific research and industry.
Preparation Methods
Synthetic Routes and Reaction Conditions
3-Deoxy-D-glucopyranose can be synthesized through several methods. One common approach involves the reduction of 3-keto-D-glucose. This reduction can be achieved using sodium borohydride (NaBH4) under mild conditions. Another method involves the deoxygenation of D-glucose derivatives using reagents like tributyltin hydride (Bu3SnH) in the presence of a radical initiator.
Industrial Production Methods
Industrial production of this compound typically involves the large-scale reduction of 3-keto-D-glucose. The process is optimized to ensure high yield and purity, often using continuous flow reactors and advanced purification techniques.
Chemical Reactions Analysis
Types of Reactions
3-Deoxy-D-glucopyranose undergoes various chemical reactions, including:
Oxidation: It can be oxidized to form 3-deoxy-D-gluconic acid using oxidizing agents like potassium permanganate (KMnO4).
Reduction: The compound can be further reduced to form 3-deoxy-D-glucitol using strong reducing agents like lithium aluminum hydride (LiAlH4).
Substitution: Halogenation reactions can introduce halogen atoms at specific positions, such as the formation of 3-chloro-3-deoxy-D-glucopyranose using thionyl chloride (SOCl2).
Common Reagents and Conditions
Oxidation: Potassium permanganate (KMnO4) in an aqueous medium.
Reduction: Sodium borohydride (NaBH4) or lithium aluminum hydride (LiAlH4) in anhydrous conditions.
Substitution: Thionyl chloride (SOCl2) in the presence of a base like pyridine.
Major Products
Oxidation: 3-Deoxy-D-gluconic acid.
Reduction: 3-Deoxy-D-glucitol.
Substitution: 3-Chloro-3-deoxy-D-glucopyranose.
Scientific Research Applications
3-Deoxy-D-glucopyranose has a wide range of applications in scientific research:
Chemistry: It is used as a building block in the synthesis of complex carbohydrates and glycoconjugates.
Biology: The compound is employed in studies of carbohydrate metabolism and enzyme specificity.
Medicine: It serves as a precursor for the synthesis of pharmaceutical agents and diagnostic tools.
Industry: this compound is used in the production of biodegradable polymers and as a starting material for various chemical syntheses.
Mechanism of Action
The mechanism of action of 3-Deoxy-D-glucopyranose involves its interaction with specific enzymes and receptors in biological systems. It can act as a substrate for enzymes involved in carbohydrate metabolism, leading to the formation of various metabolites. The compound’s unique structure allows it to participate in specific biochemical pathways, influencing cellular processes and metabolic functions.
Comparison with Similar Compounds
3-Deoxy-D-glucopyranose can be compared with other similar compounds such as:
2-Deoxy-D-glucose: Lacks the hydroxyl group at the second carbon, used in cancer research and as a glycolysis inhibitor.
3-Amino-3-deoxy-D-glucopyranose: Contains an amino group at the third carbon, used in the synthesis of aminoglycoside antibiotics.
3-Chloro-3-deoxy-D-glucopyranose: Contains a chlorine atom at the third carbon, used in carbohydrate chemistry and as a biochemical reagent.
The uniqueness of this compound lies in its specific deoxygenation at the third carbon, which imparts distinct chemical and biological properties compared to its analogs.
Properties
Molecular Formula |
C6H12O5 |
---|---|
Molecular Weight |
164.16 g/mol |
IUPAC Name |
(3R,5S,6R)-6-(hydroxymethyl)oxane-2,3,5-triol |
InChI |
InChI=1S/C6H12O5/c7-2-5-3(8)1-4(9)6(10)11-5/h3-10H,1-2H2/t3-,4+,5+,6?/m0/s1 |
InChI Key |
RJDIFQMDPPUATQ-JMSAOHGTSA-N |
Isomeric SMILES |
C1[C@@H]([C@H](OC([C@@H]1O)O)CO)O |
Canonical SMILES |
C1C(C(OC(C1O)O)CO)O |
Origin of Product |
United States |
Disclaimer and Information on In-Vitro Research Products
Please be aware that all articles and product information presented on BenchChem are intended solely for informational purposes. The products available for purchase on BenchChem are specifically designed for in-vitro studies, which are conducted outside of living organisms. In-vitro studies, derived from the Latin term "in glass," involve experiments performed in controlled laboratory settings using cells or tissues. It is important to note that these products are not categorized as medicines or drugs, and they have not received approval from the FDA for the prevention, treatment, or cure of any medical condition, ailment, or disease. We must emphasize that any form of bodily introduction of these products into humans or animals is strictly prohibited by law. It is essential to adhere to these guidelines to ensure compliance with legal and ethical standards in research and experimentation.