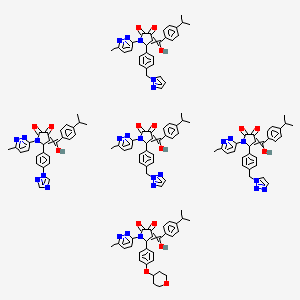
Olea europaea (olive) leaf extract
- Click on QUICK INQUIRY to receive a quote from our team of experts.
- With the quality product at a COMPETITIVE price, you can focus more on your research.
Overview
Description
Olea europaea (olive) leaf extract: is derived from the leaves of the olive tree, which is native to the Mediterranean region. The extract is rich in bioactive compounds, particularly oleuropein, which is known for its antioxidant, anti-inflammatory, and antimicrobial properties . Olive leaf extract has been used traditionally for its medicinal benefits and is now gaining scientific recognition for its potential health applications .
Preparation Methods
Synthetic Routes and Reaction Conditions: : Olive leaf extract is typically prepared through extraction methods using solvents such as ethanol, water, or hydroalcoholic mixtures . The leaves are dried and then subjected to extraction processes like Soxhlet extraction or microwave-assisted extraction . The choice of solvent and extraction method can significantly influence the yield and composition of the extract .
Industrial Production Methods: : Industrial production of olive leaf extract involves large-scale extraction processes. The dried leaves are often extracted using hydroalcoholic mixtures or ethyl acetate . The extract is then concentrated and purified to achieve the desired levels of bioactive compounds, particularly oleuropein . Advanced techniques like microwave-assisted extraction are also employed to enhance efficiency and yield .
Chemical Reactions Analysis
Types of Reactions: : Olive leaf extract undergoes various chemical reactions, including oxidation, reduction, and hydrolysis . Oleuropein, the major compound in the extract, can be hydrolyzed to produce hydroxytyrosol, which has potent antioxidant properties .
Common Reagents and Conditions: : Common reagents used in the reactions involving olive leaf extract include ethanol, water, and enzymes . The reactions are typically carried out under mild conditions to preserve the bioactive compounds .
Major Products Formed: : The major products formed from the reactions of olive leaf extract include hydroxytyrosol, oleuropein aglycone, and other phenolic compounds . These products contribute to the extract’s antioxidant and anti-inflammatory properties .
Scientific Research Applications
Chemistry: : In chemistry, olive leaf extract is studied for its antioxidant properties and its ability to scavenge free radicals . It is also used in the synthesis of bioactive compounds and as a natural preservative in food and cosmetic products .
Biology: : In biological research, olive leaf extract is investigated for its antimicrobial and antiviral activities . It has shown potential in inhibiting the growth of various pathogens, including bacteria and viruses .
Medicine: : Olive leaf extract is widely studied for its potential therapeutic applications in medicine. It has been shown to have antihypertensive, hypoglycemic, and cardioprotective effects . Research also suggests its potential in cancer treatment due to its antiproliferative and cytotoxic activities .
Industry: : In the industrial sector, olive leaf extract is used in the production of dietary supplements, functional foods, and cosmetics . Its antioxidant properties make it a valuable ingredient in preserving the quality and extending the shelf life of products .
Mechanism of Action
The mechanism of action of olive leaf extract is primarily attributed to its high content of oleuropein and other phenolic compounds . These compounds exert their effects through various molecular pathways. Oleuropein, for instance, inhibits the activity of enzymes involved in the production of reactive oxygen species, thereby reducing oxidative stress . It also modulates the expression of genes involved in inflammation and immune response . Additionally, oleuropein and its derivatives can interact with cell membranes, enhancing their stability and protecting against damage .
Comparison with Similar Compounds
Similar Compounds: : Olive leaf extract is often compared with other natural antioxidants like ascorbic acid (vitamin C) and α-tocopherol (vitamin E) . Other similar compounds include green tea extract, grape seed extract, and resveratrol .
Uniqueness: : What sets olive leaf extract apart is its high oleuropein content, which is not commonly found in other natural extracts . Oleuropein’s unique combination of antioxidant, anti-inflammatory, and antimicrobial properties makes olive leaf extract a versatile and potent bioactive compound .
Properties
CAS No. |
8060-29-5 |
---|---|
Molecular Formula |
C142H134N26O17 |
Molecular Weight |
2476.7 g/mol |
IUPAC Name |
4-[hydroxy-(4-propan-2-ylphenyl)methylidene]-1-(6-methylpyridazin-3-yl)-5-[4-(oxan-4-yloxy)phenyl]pyrrolidine-2,3-dione;4-[hydroxy-(4-propan-2-ylphenyl)methylidene]-1-(6-methylpyridazin-3-yl)-5-[4-(pyrazol-1-ylmethyl)phenyl]pyrrolidine-2,3-dione;4-[hydroxy-(4-propan-2-ylphenyl)methylidene]-1-(6-methylpyridazin-3-yl)-5-[4-(triazol-1-ylmethyl)phenyl]pyrrolidine-2,3-dione;4-[hydroxy-(4-propan-2-ylphenyl)methylidene]-1-(6-methylpyridazin-3-yl)-5-[4-(triazol-2-ylmethyl)phenyl]pyrrolidine-2,3-dione;4-[hydroxy-(4-propan-2-ylphenyl)methylidene]-1-(6-methylpyridazin-3-yl)-5-[4-(1,2,4-triazol-1-yl)phenyl]pyrrolidine-2,3-dione |
InChI |
InChI=1S/C30H31N3O5.C29H27N5O3.2C28H26N6O3.C27H24N6O3/c1-18(2)20-5-7-22(8-6-20)28(34)26-27(33(30(36)29(26)35)25-13-4-19(3)31-32-25)21-9-11-23(12-10-21)38-24-14-16-37-17-15-24;1-18(2)21-10-12-23(13-11-21)27(35)25-26(22-8-6-20(7-9-22)17-33-16-4-15-30-33)34(29(37)28(25)36)24-14-5-19(3)31-32-24;1-17(2)20-9-11-22(12-10-20)26(35)24-25(21-7-5-19(6-8-21)16-33-15-14-29-32-33)34(28(37)27(24)36)23-13-4-18(3)30-31-23;1-17(2)20-9-11-22(12-10-20)26(35)24-25(21-7-5-19(6-8-21)16-33-29-14-15-30-33)34(28(37)27(24)36)23-13-4-18(3)31-32-23;1-16(2)18-5-7-20(8-6-18)25(34)23-24(19-9-11-21(12-10-19)32-15-28-14-29-32)33(27(36)26(23)35)22-13-4-17(3)30-31-22/h4-13,18,24,27,34H,14-17H2,1-3H3;4-16,18,26,35H,17H2,1-3H3;2*4-15,17,25,35H,16H2,1-3H3;4-16,24,34H,1-3H3 |
InChI Key |
CNPGVIACQIHOGP-UHFFFAOYSA-N |
Canonical SMILES |
CC1=NN=C(C=C1)N2C(C(=C(C3=CC=C(C=C3)C(C)C)O)C(=O)C2=O)C4=CC=C(C=C4)CN5C=CC=N5.CC1=NN=C(C=C1)N2C(C(=C(C3=CC=C(C=C3)C(C)C)O)C(=O)C2=O)C4=CC=C(C=C4)CN5C=CN=N5.CC1=NN=C(C=C1)N2C(C(=C(C3=CC=C(C=C3)C(C)C)O)C(=O)C2=O)C4=CC=C(C=C4)CN5N=CC=N5.CC1=NN=C(C=C1)N2C(C(=C(C3=CC=C(C=C3)C(C)C)O)C(=O)C2=O)C4=CC=C(C=C4)N5C=NC=N5.CC1=NN=C(C=C1)N2C(C(=C(C3=CC=C(C=C3)C(C)C)O)C(=O)C2=O)C4=CC=C(C=C4)OC5CCOCC5 |
Origin of Product |
United States |
Disclaimer and Information on In-Vitro Research Products
Please be aware that all articles and product information presented on BenchChem are intended solely for informational purposes. The products available for purchase on BenchChem are specifically designed for in-vitro studies, which are conducted outside of living organisms. In-vitro studies, derived from the Latin term "in glass," involve experiments performed in controlled laboratory settings using cells or tissues. It is important to note that these products are not categorized as medicines or drugs, and they have not received approval from the FDA for the prevention, treatment, or cure of any medical condition, ailment, or disease. We must emphasize that any form of bodily introduction of these products into humans or animals is strictly prohibited by law. It is essential to adhere to these guidelines to ensure compliance with legal and ethical standards in research and experimentation.