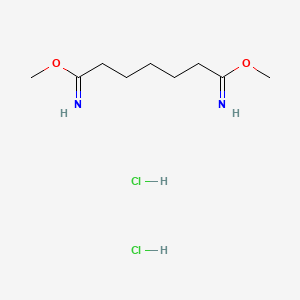
Dimethyl pimelimidate dihydrochloride
- Click on QUICK INQUIRY to receive a quote from our team of experts.
- With the quality product at a COMPETITIVE price, you can focus more on your research.
Overview
Description
Dimethyl pimelimidate dihydrochloride is an organic chemical compound with two functional imidate groups. It is commonly available as a more stable dihydrochloride salt. This compound is known for its ability to bind free amino groups within a pH range of 7.0-10.0, forming amidine bonds . It is primarily used as a bifunctional coupling reagent to link proteins, making it a valuable tool in biochemical research and industrial applications .
Mechanism of Action
Target of Action
Dimethyl pimelimidate dihydrochloride (DMP) is a homobifunctional cross-linking reagent . Its primary targets are proteins , specifically those containing primary amines . These amines are common in proteins, found in the side chains of lysine residues and the N-termini of proteins .
Mode of Action
DMP interacts with its targets by reacting with primary amines in the pH range 7.0-10.0 to form amidine bonds . This reaction allows DMP to create links between proteins, effectively crosslinking them .
Biochemical Pathways
The primary biochemical pathway affected by DMP is protein interaction and modification . By crosslinking proteins, DMP can alter the structure and function of the proteins, affecting their interactions with other molecules and their roles within cells .
Pharmacokinetics
It’s important to note that dmp is readily hydrolyzed at neutral ph , which could influence its stability and bioavailability in biological systems.
Result of Action
The molecular and cellular effects of DMP’s action largely depend on the specific proteins it crosslinks. For example, DMP has been used in chromatin immunoprecipitation experiments (ChIP) to enhance signal . In these experiments, DMP can crosslink DNA-binding proteins to DNA, allowing for the isolation and identification of these proteins .
Action Environment
The action of DMP can be influenced by environmental factors such as pH. DMP reacts with primary amines in the pH range 7.0-10.0 , and it is readily hydrolyzed at neutral pH . Therefore, the pH of the environment can significantly impact the efficacy and stability of DMP.
Biochemical Analysis
Biochemical Properties
Dimethyl pimelimidate dihydrochloride plays a crucial role in biochemical reactions by acting as a cross-linking agent. It typically reacts with primary amines in the pH range of 7.0 to 10.0 to form amidine bonds . This compound is used to link proteins, facilitating the study of protein-protein interactions. For example, it is often employed in the preparation of antibody affinity columns, where it couples antibodies to Protein A or Protein G-agarose . The amidine linkage formed by this compound preserves the original positive charge of the primary amine, which is essential for maintaining the functionality of the linked proteins .
Cellular Effects
This compound influences various cellular processes by modifying proteins and their interactions. It has been used in chromatin immunoprecipitation experiments to enhance signal detection . This compound can affect cell signaling pathways, gene expression, and cellular metabolism by cross-linking proteins involved in these processes. For instance, it can alter the function of signaling proteins by stabilizing their interactions, leading to changes in downstream signaling events .
Molecular Mechanism
The molecular mechanism of this compound involves its ability to bind free amino groups at a pH range of 7.0 to 10.0, forming stable amidine bonds . This binding interaction is crucial for its role as a cross-linking agent. By linking proteins, this compound can stabilize protein complexes and facilitate the study of protein interactions. Additionally, it can influence enzyme activity by modifying the structure of enzymes through cross-linking, leading to either inhibition or activation of enzymatic functions .
Temporal Effects in Laboratory Settings
In laboratory settings, the effects of this compound can change over time due to its stability and degradation properties. This compound is readily hydrolyzed at neutral pH, which can affect its long-term stability . Studies have shown that this compound can maintain its cross-linking activity for extended periods when stored under appropriate conditions, such as at -20°C . Prolonged exposure to neutral or acidic conditions can lead to degradation, reducing its effectiveness in cross-linking reactions .
Dosage Effects in Animal Models
The effects of this compound vary with different dosages in animal models. At lower doses, it can effectively cross-link proteins without causing significant toxicity . At higher doses, it may exhibit toxic or adverse effects, such as irritation to the eyes, skin, mucous membranes, and upper respiratory tract . It is essential to determine the optimal dosage to achieve the desired cross-linking effects while minimizing potential toxicity in animal studies .
Metabolic Pathways
This compound is involved in metabolic pathways that include interactions with enzymes and cofactors. It primarily reacts with primary amines to form amidine bonds, which can affect metabolic flux and metabolite levels . By cross-linking proteins involved in metabolic pathways, this compound can influence the activity and regulation of these pathways .
Transport and Distribution
Within cells and tissues, this compound is transported and distributed through interactions with transporters and binding proteins . Its localization and accumulation can be influenced by these interactions, affecting its availability for cross-linking reactions. The compound’s ability to form stable amidine bonds with primary amines ensures its effective distribution within cellular environments .
Subcellular Localization
This compound exhibits specific subcellular localization, which can impact its activity and function. It can be directed to specific compartments or organelles through targeting signals or post-translational modifications . This localization is essential for its role in cross-linking proteins within particular cellular contexts, such as chromatin immunoprecipitation experiments .
Preparation Methods
Synthetic Routes and Reaction Conditions: Dimethyl pimelimidate dihydrochloride is synthesized through the reaction of pimelic acid with methanol in the presence of a dehydrating agent. The reaction typically involves the formation of an ester intermediate, which is then converted to the diimidate form. The final product is obtained as a dihydrochloride salt to enhance its stability .
Industrial Production Methods: In industrial settings, the production of this compound involves large-scale esterification and subsequent conversion to the diimidate form. The process is optimized for high yield and purity, ensuring the compound meets the stringent requirements for biochemical applications .
Chemical Reactions Analysis
Types of Reactions: Dimethyl pimelimidate dihydrochloride primarily undergoes substitution reactions, where it reacts with primary amines to form amidine bonds . This reaction is facilitated by the presence of imidate groups at each end of the molecule.
Common Reagents and Conditions: The reaction with primary amines occurs optimally at alkaline pH values (pH 7.0-10.0). Common reagents used in these reactions include primary amines and buffers to maintain the desired pH .
Major Products: The major product formed from the reaction of this compound with primary amines is an amidine bond, which retains the net charge character of the protein primary amine to which it reacts .
Scientific Research Applications
Dimethyl pimelimidate dihydrochloride has a wide range of applications in scientific research:
Comparison with Similar Compounds
Dimethyl pimelimidate dihydrochloride is part of a family of homobifunctional cross-linking reagents, which includes dimethyl adipimidate and dimethyl suberimidate .
Dimethyl adipimidate: This compound has a shorter spacer arm compared to this compound, making it suitable for cross-linking reactions that require closer proximity between reactive groups.
Dimethyl suberimidate: With a longer spacer arm, this compound is used for cross-linking reactions that require greater distance between reactive groups.
The uniqueness of this compound lies in its mid-length spacer arm, which provides a balance between flexibility and stability in cross-linking reactions .
Properties
CAS No. |
58537-94-3 |
---|---|
Molecular Formula |
C9H19ClN2O2 |
Molecular Weight |
222.71 g/mol |
IUPAC Name |
dimethyl heptanediimidate;hydrochloride |
InChI |
InChI=1S/C9H18N2O2.ClH/c1-12-8(10)6-4-3-5-7-9(11)13-2;/h10-11H,3-7H2,1-2H3;1H |
InChI Key |
HUYMOELLLZYLES-UHFFFAOYSA-N |
SMILES |
COC(=N)CCCCCC(=N)OC.Cl.Cl |
Canonical SMILES |
COC(=N)CCCCCC(=N)OC.Cl |
Pictograms |
Irritant |
Origin of Product |
United States |
Retrosynthesis Analysis
AI-Powered Synthesis Planning: Our tool employs the Template_relevance Pistachio, Template_relevance Bkms_metabolic, Template_relevance Pistachio_ringbreaker, Template_relevance Reaxys, Template_relevance Reaxys_biocatalysis model, leveraging a vast database of chemical reactions to predict feasible synthetic routes.
One-Step Synthesis Focus: Specifically designed for one-step synthesis, it provides concise and direct routes for your target compounds, streamlining the synthesis process.
Accurate Predictions: Utilizing the extensive PISTACHIO, BKMS_METABOLIC, PISTACHIO_RINGBREAKER, REAXYS, REAXYS_BIOCATALYSIS database, our tool offers high-accuracy predictions, reflecting the latest in chemical research and data.
Strategy Settings
Precursor scoring | Relevance Heuristic |
---|---|
Min. plausibility | 0.01 |
Model | Template_relevance |
Template Set | Pistachio/Bkms_metabolic/Pistachio_ringbreaker/Reaxys/Reaxys_biocatalysis |
Top-N result to add to graph | 6 |
Feasible Synthetic Routes
Q1: What is Dimethyl pimelimidate dihydrochloride used for in the context of the provided research papers?
A1: this compound is used as a crosslinking agent to covalently attach antibodies to solid supports. This is a crucial step in immunoaffinity purification techniques. For example, in one study, this compound was used to covalently link a monoclonal antibody (MAb 6E52D9) to a Streamline rProtein A adsorbent. This created an immunoaffinity matrix for the purification of Enterocytozoon bieneusi spores from human stool samples.
Q2: How does this compound facilitate the crosslinking process?
A2: this compound is a homobifunctional crosslinker, meaning it has two reactive groups capable of forming bonds with amine groups. In the context of the research papers, it reacts with primary amine groups present on both the antibody and the Protein G-coated beads. This reaction forms stable amide bonds, effectively immobilizing the antibodies onto the solid support.
Q3: Why is the crosslinking step with this compound important in these studies?
A3: The covalent crosslinking ensures that the antibodies remain bound to the solid support during subsequent washing steps, which are necessary to remove unbound materials. This is crucial for achieving a high purity of the target molecule. In the case of the Enterocytozoon bieneusi study, this allowed for the efficient removal of contaminants from human stool samples and the isolation of highly pure spores. Similarly, in the other study, it facilitated the capture and analysis of specific tumor antigens from complex protein mixtures.
Disclaimer and Information on In-Vitro Research Products
Please be aware that all articles and product information presented on BenchChem are intended solely for informational purposes. The products available for purchase on BenchChem are specifically designed for in-vitro studies, which are conducted outside of living organisms. In-vitro studies, derived from the Latin term "in glass," involve experiments performed in controlled laboratory settings using cells or tissues. It is important to note that these products are not categorized as medicines or drugs, and they have not received approval from the FDA for the prevention, treatment, or cure of any medical condition, ailment, or disease. We must emphasize that any form of bodily introduction of these products into humans or animals is strictly prohibited by law. It is essential to adhere to these guidelines to ensure compliance with legal and ethical standards in research and experimentation.