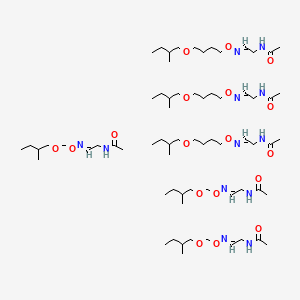
Agarase
- Click on QUICK INQUIRY to receive a quote from our team of experts.
- With the quality product at a COMPETITIVE price, you can focus more on your research.
Overview
Description
Agarase is an enzyme that catalyzes the hydrolysis of agar, a polysaccharide found in the cell walls of red algae. Agar is composed of repetitive units of β-D-galactose and 3,6-anhydro-α-L-galactose. This compound is classified into two types based on the cleavage pattern: α-agarase and β-agarase. α-Agarase cleaves α-1,3 linkages to produce agarooligosaccharides, while β-agarase cleaves β-1,4 linkages to produce neoagarooligosaccharides .
Preparation Methods
Synthetic Routes and Reaction Conditions: Agarase is typically produced by microorganisms such as bacteria found in seawater and marine sediments. The enzyme can be isolated and purified from these microorganisms through various biochemical techniques. The production process involves culturing the bacteria in a suitable medium, followed by enzyme extraction and purification using methods such as ammonium sulfate precipitation, dialysis, and chromatography .
Industrial Production Methods: Industrial production of this compound involves large-scale fermentation processes. Marine bacteria such as Pseudoalteromonas, Vibrio, and Flammeovirga are commonly used for this purpose. The fermentation process is optimized to enhance enzyme yield, and the enzyme is subsequently purified using techniques like ultrafiltration and ion-exchange chromatography .
Chemical Reactions Analysis
Types of Reactions: Agarase primarily catalyzes the hydrolysis of agarose, breaking down the polysaccharide into smaller oligosaccharides. The enzyme does not typically undergo oxidation, reduction, or substitution reactions. Instead, it specifically targets the glycosidic bonds within the agarose structure .
Common Reagents and Conditions: The hydrolysis reaction catalyzed by this compound requires specific conditions, including optimal pH and temperature. For instance, α-agarase from Catenovulum maritimus STB14 operates effectively at a temperature of 42°C and a pH of 7.5 . Common reagents used in the reaction include buffers to maintain the pH and salts to provide necessary ions for enzyme activity.
Major Products: The primary products of this compound-catalyzed hydrolysis are agarooligosaccharides and neoagarooligosaccharides. These oligosaccharides have various degrees of polymerization and are valuable for their biological activities .
Scientific Research Applications
Agarase has a wide range of applications in scientific research and industry:
Biology: The enzyme is employed to recover DNA from agarose gels, a common technique in molecular biology.
Industry: this compound is used in the food industry to produce oligosaccharides with prebiotic properties.
Mechanism of Action
Agarase exerts its effects by hydrolyzing the glycosidic bonds in agarose. The enzyme binds to the substrate and catalyzes the cleavage of the glycosidic linkages, resulting in the formation of smaller oligosaccharides. The catalytic mechanism involves conserved residues in the active site of the enzyme, which facilitate the hydrolysis reaction . Molecular dynamics simulations have provided insights into the thermostability and catalytic efficiency of this compound .
Comparison with Similar Compounds
Agarase is unique in its ability to specifically hydrolyze agarose. Similar enzymes include:
Cellulase: Hydrolyzes cellulose into glucose.
Xylanase: Breaks down xylan into xylose.
Chitinase: Degrades chitin into N-acetylglucosamine.
Compared to these enzymes, this compound is specialized for agarose hydrolysis and produces unique oligosaccharides with distinct biological activities .
Biological Activity
Agarase is an enzyme that catalyzes the hydrolysis of agar, a polysaccharide derived from red algae, into smaller oligosaccharides. This enzyme is categorized into two main types: α-agarase and β-agarase , which differ based on their cleavage patterns. α-Agarases cleave α-1,3-glycosidic bonds, producing agarooligosaccharides, while β-agarases cleave β-1,4-glycosidic bonds to yield neoagarooligosaccharides (NAOS) . The biological activity of this compound and its products has significant implications in various fields, including biotechnology, medicine, and food science.
This compound acts on the structure of agarose, which is composed of repeating units of β-D-galactose and 3,6-anhydro-α-L-galactose. The enzymatic breakdown results in oligosaccharides that exhibit various biological activities. The hydrolysis products can inhibit inflammatory markers such as nitric oxide (NO), interleukins 6 (IL-6), and tumor necrosis factor α (TNF-α), indicating potential anti-inflammatory properties .
Applications in Biotechnology and Medicine
- Production of Oligosaccharides : Agarases are used to produce bioactive oligosaccharides that have health benefits, such as prebiotic effects and immune modulation .
- Industrial Uses : The enzyme's ability to hydrolyze agar makes it valuable in the food industry for improving texture and stability in products like jellies and desserts.
- Pharmaceutical Applications : Agarases can be utilized to enhance drug delivery systems by modifying the viscosity of gels used in formulations .
Case Study 1: Anti-inflammatory Effects
In a study involving recombinant β-agarase (Aga1904) from Antarctic macroalgae, the agaro-oligosaccharides produced were shown to significantly reduce inflammatory markers in LPS-stimulated RAW264.7 macrophages. The results indicated that neoagarobiose was particularly effective in inhibiting the production of NO and TNF-α .
Case Study 2: Industrial Enzyme Production
Research on Bacillus subtilis PI demonstrated improved production of this compound through optimization techniques involving various substrates such as peptone and yeast extract. This study highlighted the importance of environmental factors on enzyme yield and activity .
Comparative Analysis of Agarases
The following table summarizes key characteristics of different agarases:
Type | Source | Cleavage Pattern | Main Products | Optimal Conditions |
---|---|---|---|---|
α-Agarase | Various bacteria | α-1,3 linkages | Agarooligosaccharides | pH 6.0, 37°C |
β-Agarase | Alteromonas sp. | β-1,4 linkages | Neoagarooligosaccharides | pH 8.0, 30°C |
Recombinant | E. coli | β-1,4 linkages | Neoagarobiose | pH 7.0, 37°C |
Properties
CAS No. |
37288-57-6 |
---|---|
Molecular Formula |
C69H138N12O18 |
Molecular Weight |
1423.9 g/mol |
IUPAC Name |
N-[2-[4-(2-methylbutoxy)butoxyimino]ethyl]acetamide;N-[2-(2-methylbutoxymethoxyimino)ethyl]acetamide |
InChI |
InChI=1S/3C13H26N2O3.3C10H20N2O3/c3*1-4-12(2)11-17-9-5-6-10-18-15-8-7-14-13(3)16;3*1-4-9(2)7-14-8-15-12-6-5-11-10(3)13/h3*8,12H,4-7,9-11H2,1-3H3,(H,14,16);3*6,9H,4-5,7-8H2,1-3H3,(H,11,13) |
InChI Key |
PQTNFQUUTNQHGS-UHFFFAOYSA-N |
Canonical SMILES |
CCC(C)COCCCCON=CCNC(=O)C.CCC(C)COCCCCON=CCNC(=O)C.CCC(C)COCCCCON=CCNC(=O)C.CCC(C)COCON=CCNC(=O)C.CCC(C)COCON=CCNC(=O)C.CCC(C)COCON=CCNC(=O)C |
Origin of Product |
United States |
Disclaimer and Information on In-Vitro Research Products
Please be aware that all articles and product information presented on BenchChem are intended solely for informational purposes. The products available for purchase on BenchChem are specifically designed for in-vitro studies, which are conducted outside of living organisms. In-vitro studies, derived from the Latin term "in glass," involve experiments performed in controlled laboratory settings using cells or tissues. It is important to note that these products are not categorized as medicines or drugs, and they have not received approval from the FDA for the prevention, treatment, or cure of any medical condition, ailment, or disease. We must emphasize that any form of bodily introduction of these products into humans or animals is strictly prohibited by law. It is essential to adhere to these guidelines to ensure compliance with legal and ethical standards in research and experimentation.