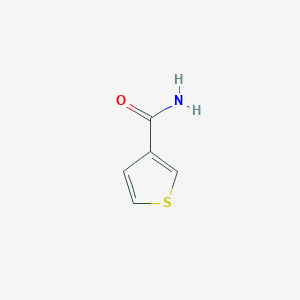
Thiophene-3-carboxamide
Overview
Description
Thiophene-3-carboxamide is a heterocyclic compound containing a sulfur atom in its five-membered ring structure. It is a derivative of thiophene, which is known for its aromatic properties and significant role in medicinal chemistry. This compound is recognized for its potential therapeutic applications and is a subject of interest in various scientific research fields.
Preparation Methods
Synthetic Routes and Reaction Conditions: Thiophene-3-carboxamide can be synthesized through several methods. One common approach involves the reaction of thiophene-3-carboxylic acid with ammonia or an amine under appropriate conditions. The reaction typically requires a dehydrating agent such as thionyl chloride or phosphorus trichloride to facilitate the formation of the amide bond.
Industrial Production Methods: In industrial settings, the synthesis of this compound may involve large-scale reactions using automated reactors. The process often includes steps such as purification through recrystallization or chromatography to ensure the compound’s purity and quality.
Chemical Reactions Analysis
Types of Reactions: Thiophene-3-carboxamide undergoes various chemical reactions, including:
Oxidation: It can be oxidized to form sulfoxides or sulfones.
Reduction: Reduction reactions can convert it to thiophene-3-carboxylamine.
Substitution: Electrophilic substitution reactions can occur at the thiophene ring, leading to various substituted derivatives.
Common Reagents and Conditions:
Oxidation: Reagents such as hydrogen peroxide or m-chloroperbenzoic acid.
Reduction: Reagents like lithium aluminum hydride or sodium borohydride.
Substitution: Reagents such as halogens, nitrating agents, or sulfonating agents.
Major Products:
Oxidation: this compound sulfoxide or sulfone.
Reduction: Thiophene-3-carboxylamine.
Substitution: Various substituted this compound derivatives.
Scientific Research Applications
Thiophene-3-carboxamide and its derivatives have a variety of applications in scientific research, particularly in medicinal chemistry and pharmacology. These applications stem from their diverse biological activities, including anti-inflammatory, anti-cancer, and antihypertensive effects .
Scientific Research Applications
Anti-inflammatory Properties:
- Thiophene carboxamide derivatives are known for their anti-inflammatory properties . They can inhibit the IKK-2 enzyme, which is vital in inducing pro-inflammatory genes. These genes include cytokines, chemokines, and cell adhesion molecules .
- These compounds can be used to treat inflammatory conditions such as rheumatoid arthritis, osteoarthritis, inflammatory bowel disease, asthma, and psoriasis .
Anti-cancer Agents:
- Benzo[b]thiophene-3-carboxylic acid 1,1-dioxide derivatives have shown potential as anticancer agents . These derivatives can inhibit the proliferation, migration, and invasion of cancer cells and promote their apoptosis .
- They target the RhoA/ROCK pathway, which is important in cancer development .
- Thiophene carboxamides can also act as dual vascular endothelial growth factor receptors (VEGFRs) and β-tubulin polymerization inhibitors, which can increase cytotoxicity towards tumor cells and decrease cancer cells' resistance to apoptosis .
Antihypertensive Effects:
- Certain thiophene-3-carboxamides have demonstrated antihypertensive effects . For example, they can attenuate hypertension and cardiac hypertrophy in hypertensive rats .
- These compounds can also inhibit protein kinase A (PKA) levels, which are involved in hypertension .
Nuclear Factor-κB (NF-κB) Inhibitors:
- Thiophene carboxamide derivatives can inhibit NF-κB, a transcription factor that induces and coordinates the expression of pro-inflammatory genes .
- Inhibiting NF-κB can help in treating various diseases, including inflammatory diseases, immune complex diseases, and cancer .
Other Applications:
Mechanism of Action
The mechanism of action of thiophene-3-carboxamide involves its interaction with specific molecular targets. For instance, it may inhibit certain enzymes or receptors, leading to its observed biological effects. The exact pathways and targets can vary depending on the specific application and derivative of the compound.
Comparison with Similar Compounds
- Thiophene-2-carboxamide
- Thiophene-3-carboxylic acid
- Thiophene-2-carboxylic acid
Biological Activity
Thiophene-3-carboxamide is a compound that has garnered attention in recent years due to its diverse biological activities, particularly in the fields of oncology and antimicrobial research. This article delves into the biological activity of this compound, highlighting its anticancer properties, mechanisms of action, and potential therapeutic applications.
Overview of this compound
This compound (TCA) is a derivative of thiophene, a five-membered aromatic ring containing sulfur. The carboxamide functional group enhances the compound's solubility and reactivity, making it a valuable scaffold for drug design. Various derivatives of TCA have been synthesized to explore their biological potential.
Recent studies have demonstrated that this compound derivatives exhibit significant anticancer activity by targeting various cellular mechanisms. For instance, one study identified two potent derivatives, 2b and 2e , which showed IC50 values of 5.46 µM and 12.58 µM against Hep3B hepatocellular carcinoma cells, respectively . These compounds disrupt tubulin dynamics, mimicking the action of established chemotherapeutics like Combretastatin A-4 (CA-4) by binding to the colchicine site on tubulin .
Case Studies
- JCI-20679 : This novel this compound analog exhibited potent antitumor activity by inhibiting mitochondrial complex I. In xenograft models, JCI-20679 demonstrated significant tumor growth inhibition across a panel of 39 human cancer cell lines . The compound's unique mechanism was characterized by a distinct growth inhibition profile that did not align with typical anticancer agents, suggesting a novel pathway for therapeutic intervention.
- NK-128 : Another derivative evaluated in chronic myeloid leukemia (CML) models showed enhanced efficacy when combined with imatinib. This combination therapy resulted in significant tumor volume reduction in xenograft models, indicating that TCA derivatives can potentiate the effects of existing therapies .
Antimicrobial Activity
This compound derivatives have also been studied for their antibacterial and antifungal properties. Research has shown that certain compounds exhibit effective antibacterial activity against various strains, including those resistant to conventional antibiotics . For instance, two biologically active derivatives demonstrated notable antibacterial effects, highlighting their potential as new antimicrobial agents.
Structure-Activity Relationship (SAR)
The biological activity of this compound is highly dependent on its structural modifications. Studies have explored various substitutions on the thiophene ring and carboxamide group to optimize potency and selectivity. For example, the introduction of halogens or alkyl groups has been shown to enhance anticancer activity while minimizing toxicity .
Compound | IC50 (µM) | Target | Activity |
---|---|---|---|
2b | 5.46 | Hep3B | Anticancer |
2e | 12.58 | Hep3B | Anticancer |
JCI-20679 | N/A | Mitochondrial Complex I | Antitumor |
NK-128 | N/A | CML | Antileukemic |
Q & A
Basic Research Questions
Q. What synthetic routes are effective for synthesizing thiophene-3-carboxamide derivatives?
Methodological Answer: this compound derivatives are typically synthesized via coupling reactions using carbodiimide-based reagents. A validated protocol involves reacting carboxylic acid derivatives with amines using EDC (1-ethyl-3-(3-dimethylaminopropyl)carbodiimide) and HOBt (hydroxybenzotriazole) as coupling agents, with DIEA (N,N-diisopropylethylamine) as a base in DMF at room temperature . Purity is confirmed using HPLC and elemental analysis, while structural characterization employs NMR and mass spectrometry .
Q. What structural features of this compound derivatives are critical for JNK inhibition?
Methodological Answer: The 3-carboxamide group on the thiophene ring is essential for JNK1 inhibition. Substitutions at the 2-position (e.g., naphthyl or fluorophenyl groups) enhance binding affinity, while replacing the thiophene core with a benzene ring or modifying the 5-position abolishes activity . Di-substitution at the 4- and 5-positions (e.g., methyl groups) improves metabolic stability by reducing oxidative degradation .
Q. What standard assays are used to evaluate the kinase inhibition and stability of this compound derivatives?
Methodological Answer:
- Kinase Activity : TR-FRET (time-resolved fluorescence resonance energy transfer) assays, such as the LanthaScreen™ platform, measure JNK1 inhibition by quantifying phosphorylation of ATF2 or c-Jun substrates .
- Plasma Stability : Compounds are incubated in rat plasma at 37°C, followed by LC-MS analysis to calculate remaining parent compound percentages .
- Metabolic Stability : Rat liver microsomes (RLM) are used with NADPH cofactors to assess degradation rates over 60 minutes .
Advanced Research Questions
Q. How can contradictory data between in vitro stability (plasma) and metabolic degradation (microsomes) be resolved for this compound derivatives?
Methodological Answer: Discrepancies arise due to differences in enzymatic environments. For example, compounds stable in plasma (e.g., 85% degradation in microsomes vs. minimal plasma degradation) suggest susceptibility to cytochrome P450-mediated oxidation . Mitigation strategies include:
- Introducing electron-withdrawing substituents (e.g., fluorine) to reduce oxidative metabolism.
- Using deuterated analogs to slow C-H bond cleavage.
- Validating with human liver microsomes (HLM) for translational relevance .
Q. What experimental approaches confirm dual inhibition of JNK via ATP and JIP-binding sites?
Methodological Answer: Dual inhibition is validated through:
- Isothermal Titration Calorimetry (ITC) : Measures binding affinity (Kd) of compounds to JNK2 in the presence/absence of ATPγS or pepJIP1. A significant reduction in binding under competitive conditions confirms dual-site interaction .
- Molecular Docking : Flexible ligand docking (e.g., using GOLD software) identifies binding poses in both ATP and D-domain pockets. For example, compound 25 showed a Kd of 640 nM for JNK2, with binding attenuated by ATPγS or pepJIP1 .
Q. How can metabolic stability of this compound derivatives be optimized without compromising kinase inhibition?
Methodological Answer:
- Structure-Activity Relationship (SAR) : Di-substitution at the 4- and 5-positions (e.g., 4,5-dimethyl groups) reduces metabolic oxidation while maintaining JNK1 inhibition (IC50 = 26 μM) .
- Prodrug Design : Masking polar groups (e.g., esterification of carboxamide) improves solubility and delays degradation.
- Species-Specific Profiling : Cross-testing in human vs. rat microsomes identifies species-specific metabolic pathways .
Q. What cell-based assays validate the suppression of c-Jun phosphorylation by this compound derivatives?
Methodological Answer:
- LanthaScreen™ Cellular Assay : HeLa cells stably expressing GFP-c-Jun (1–79) are treated with compounds, lysed, and analyzed via TR-FRET. Phosphorylation is quantified using a terbium-labeled anti-phospho-c-Jun antibody, with emission ratios (520/495 nm) indicating inhibition efficacy .
- Dose-Response Curves : IC50 values derived from these assays correlate with in vitro kinase data, ensuring cellular permeability and target engagement .
Q. How do molecular modeling studies guide the optimization of this compound derivatives?
Methodological Answer:
- Docking Simulations : Using JNK1 crystal structures (PDB: 3E7O), compounds are flexibly docked to prioritize substituents that fill hydrophobic pockets (e.g., naphthyl groups at the 2-position) .
- Free Energy Calculations : MM-GBSA scoring predicts binding affinities, aligning with experimental Kd values. For example, fluorophenyl substitutions improve van der Waals interactions, reducing entropic penalties .
Properties
IUPAC Name |
thiophene-3-carboxamide | |
---|---|---|
Source | PubChem | |
URL | https://pubchem.ncbi.nlm.nih.gov | |
Description | Data deposited in or computed by PubChem | |
InChI |
InChI=1S/C5H5NOS/c6-5(7)4-1-2-8-3-4/h1-3H,(H2,6,7) | |
Source | PubChem | |
URL | https://pubchem.ncbi.nlm.nih.gov | |
Description | Data deposited in or computed by PubChem | |
InChI Key |
DAUYIKBTMNZABP-UHFFFAOYSA-N | |
Source | PubChem | |
URL | https://pubchem.ncbi.nlm.nih.gov | |
Description | Data deposited in or computed by PubChem | |
Canonical SMILES |
C1=CSC=C1C(=O)N | |
Source | PubChem | |
URL | https://pubchem.ncbi.nlm.nih.gov | |
Description | Data deposited in or computed by PubChem | |
Molecular Formula |
C5H5NOS | |
Source | PubChem | |
URL | https://pubchem.ncbi.nlm.nih.gov | |
Description | Data deposited in or computed by PubChem | |
DSSTOX Substance ID |
DTXSID70458610 | |
Record name | Thiophene-3-carboxamide | |
Source | EPA DSSTox | |
URL | https://comptox.epa.gov/dashboard/DTXSID70458610 | |
Description | DSSTox provides a high quality public chemistry resource for supporting improved predictive toxicology. | |
Molecular Weight |
127.17 g/mol | |
Source | PubChem | |
URL | https://pubchem.ncbi.nlm.nih.gov | |
Description | Data deposited in or computed by PubChem | |
CAS No. |
51460-47-0 | |
Record name | Thiophene-3-carboxamide | |
Source | EPA DSSTox | |
URL | https://comptox.epa.gov/dashboard/DTXSID70458610 | |
Description | DSSTox provides a high quality public chemistry resource for supporting improved predictive toxicology. | |
Record name | Thiophene-3-carboxylic acid amide | |
Source | European Chemicals Agency (ECHA) | |
URL | https://echa.europa.eu/information-on-chemicals | |
Description | The European Chemicals Agency (ECHA) is an agency of the European Union which is the driving force among regulatory authorities in implementing the EU's groundbreaking chemicals legislation for the benefit of human health and the environment as well as for innovation and competitiveness. | |
Explanation | Use of the information, documents and data from the ECHA website is subject to the terms and conditions of this Legal Notice, and subject to other binding limitations provided for under applicable law, the information, documents and data made available on the ECHA website may be reproduced, distributed and/or used, totally or in part, for non-commercial purposes provided that ECHA is acknowledged as the source: "Source: European Chemicals Agency, http://echa.europa.eu/". Such acknowledgement must be included in each copy of the material. ECHA permits and encourages organisations and individuals to create links to the ECHA website under the following cumulative conditions: Links can only be made to webpages that provide a link to the Legal Notice page. | |
Retrosynthesis Analysis
AI-Powered Synthesis Planning: Our tool employs the Template_relevance Pistachio, Template_relevance Bkms_metabolic, Template_relevance Pistachio_ringbreaker, Template_relevance Reaxys, Template_relevance Reaxys_biocatalysis model, leveraging a vast database of chemical reactions to predict feasible synthetic routes.
One-Step Synthesis Focus: Specifically designed for one-step synthesis, it provides concise and direct routes for your target compounds, streamlining the synthesis process.
Accurate Predictions: Utilizing the extensive PISTACHIO, BKMS_METABOLIC, PISTACHIO_RINGBREAKER, REAXYS, REAXYS_BIOCATALYSIS database, our tool offers high-accuracy predictions, reflecting the latest in chemical research and data.
Strategy Settings
Precursor scoring | Relevance Heuristic |
---|---|
Min. plausibility | 0.01 |
Model | Template_relevance |
Template Set | Pistachio/Bkms_metabolic/Pistachio_ringbreaker/Reaxys/Reaxys_biocatalysis |
Top-N result to add to graph | 6 |
Feasible Synthetic Routes
Disclaimer and Information on In-Vitro Research Products
Please be aware that all articles and product information presented on BenchChem are intended solely for informational purposes. The products available for purchase on BenchChem are specifically designed for in-vitro studies, which are conducted outside of living organisms. In-vitro studies, derived from the Latin term "in glass," involve experiments performed in controlled laboratory settings using cells or tissues. It is important to note that these products are not categorized as medicines or drugs, and they have not received approval from the FDA for the prevention, treatment, or cure of any medical condition, ailment, or disease. We must emphasize that any form of bodily introduction of these products into humans or animals is strictly prohibited by law. It is essential to adhere to these guidelines to ensure compliance with legal and ethical standards in research and experimentation.