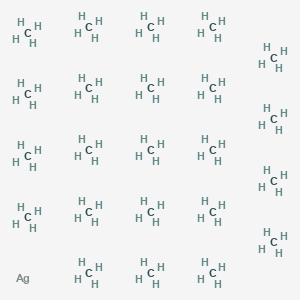
methane;silver
- Click on QUICK INQUIRY to receive a quote from our team of experts.
- With the quality product at a COMPETITIVE price, you can focus more on your research.
Overview
Description
Methane (CH₄) is the simplest hydrocarbon and a potent greenhouse gas with significant roles in energy production and environmental chemistry. Silver (Ag) and its compounds are widely studied in catalysis and material science due to their unique electronic properties and surface reactivity. The interaction between methane and silver-based materials, particularly in catalytic processes and gas storage applications, has garnered attention for optimizing energy efficiency and reducing environmental impact.
Silver-based catalysts, such as single-atom silver catalysts (e.g., Ag-HMO) and silver-decorated metal oxides (e.g., Ag/ZnO), demonstrate exceptional performance in methane conversion and photocatalytic reactions . Additionally, silver nanoparticles (Ag NPs) have been explored for their role in accelerating methane hydrate formation, a critical process for natural gas storage .
Preparation Methods
Synthetic Routes and Reaction Conditions
The preparation of methane;silver compounds typically involves the use of silver catalysts in various forms, such as silver nanoparticles or silver-exchanged zeolites. One common method is the oxidative coupling of methane (OCM) over silver catalysts. This process involves the reaction of methane with oxygen in the presence of a silver catalyst at high temperatures, typically above 1020 K . The reaction conditions are carefully controlled to optimize the yield of desired products like ethane and ethylene while minimizing the formation of unwanted by-products like carbon dioxide .
Industrial Production Methods
In industrial settings, the production of this compound compounds often involves the use of silver-exchanged zeolites. These catalysts are prepared by exchanging the cations in zeolites with silver ions, followed by calcination to activate the catalyst. The process is optimized to achieve high selectivity and yield of the desired products .
Chemical Reactions Analysis
Photocatalytic Methane Oxidation with Silver-Decorated ZnO
Silver nanoparticles (Ag NPs) deposited on zinc oxide (ZnO) enhance methane oxidation under simulated sunlight via surface plasmon resonance.
-
Reaction : CH4+O2Ag-ZnO, lightCO2+H2O
-
Performance :
-
Mechanism :
Synergistic Methane Oxidation to Methanol via Ag–Cu Dual Single-Atom Catalysts
Ag and Cu single atoms on ZSM-5 zeolite enable selective methane oxidation to methanol using H₂O₂ under mild conditions .
-
Reaction : CH4+H2O2Ag1-Cu1/ZSM-5CH3OH
-
Performance :
-
Key Features :
Room-Temperature Methane Activation with MgO/CuO/Ag Nanocomposites
A layered catalyst (MgO NPs embedded in CuO/Cu) activates methane at ambient temperatures :
-
Reaction : 2CH4MgO/CuO/AgC2H6+H2
-
Performance :
-
Mechanism :
Light-Driven Methane Coupling via Silver Nanocomposites
Ag-based nanocomposites under UV/visible light induce methane coupling to ethane :
-
Reaction : 2CH4Ag nanocomposite, lightC2H6+H2
-
Performance :
-
Mechanism :
Silver-Catalyzed C–C Bond Formation with Ethyl Diazoacetate
Silver perfluorinated indazolylborate complexes mediate methane coupling to ethyl propionate in supercritical CO₂ :
-
Reaction : CH4+N2CHCO2EtAg catalyst, scCO2CH3CH2CO2Et
-
Performance :
-
Mechanism :
Spectroscopic Insights into Silver–Methane Interactions
Ion spectroscopy reveals coordination-dependent methane activation pathways :
-
η² Coordination : Methane binds via two C–H bonds to Ag⁺, enabling H abstraction.
-
η³ Coordination : Methane binds via three C–H bonds, typical of weaker electrostatic interactions.
Comparative Analysis of Key Catalytic Systems
Scientific Research Applications
Methane;silver compounds have a wide range of scientific research applications, including:
Mechanism of Action
The mechanism by which methane;silver compounds exert their effects involves the activation of methane molecules on the surface of silver catalysts. Silver facilitates the abstraction of hydrogen atoms from methane, leading to the formation of reactive intermediates that can undergo further reactions to produce desired products . The molecular targets and pathways involved include the formation of surface-bound oxygen species that interact with methane to initiate the catalytic process .
Comparison with Similar Compounds
Comparison with Other Catalysts in Methane Oxidation
Silver-based catalysts are compared with noble metals (e.g., Pt, Pd) and transition metal oxides (e.g., CuO, Al₂O₃) in methane oxidation, a process vital for reducing unburned methane emissions.
Key Findings:
- Ag/ZnO Nanocatalysts: Under simulated sunlight, Ag-decorated ZnO achieved 85% methane conversion at 150°C, outperforming undoped ZnO (35% conversion) .
- Noble Metals on Hydroxyapatite (HAP): Pd/HAP: Highest activity at 400°C (90% CH₄ conversion). Ag/HAP: Moderate activity (60% conversion) but superior stability under lean conditions .
- Pt/ZrO₂ : Achieves complete methane oxidation at 450°C, leveraging metal-support interactions .
Table 1: Methane Oxidation Performance of Catalysts
Catalyst | Temperature (°C) | CH₄ Conversion (%) | Stability | Reference |
---|---|---|---|---|
Ag/ZnO | 150 | 85 | High (20 cycles) | |
Pd/HAP | 400 | 90 | Moderate | |
Pt/ZrO₂ | 450 | 100 | High | |
CuO Nanoparticles | 275 | 50 | Low (deactivation) |
Comparison in Methane Hydrate Formation Kinetics
Methane hydrates are clathrate structures used for gas storage. Nanoparticles like Ag, CuO, and Al₂O₃ are additives to accelerate hydrate formation.
Key Findings:
- Carbon Nanostructures: Multi-wall carbon nanotubes (MWCNTs) increase gas consumption by 56%, but Ag NPs show faster nucleation .
Table 2: Induction Time Reduction in Methane Hydrate Formation
Additive | Induction Time Reduction (%) | Pressure (MPa) | Reference |
---|---|---|---|
Ag NPs | 97 | 5.5 | |
CuO NPs | 79.4 | 5.5 | |
Al₂O₃ NPs | 70 | 7.0 | |
MWCNTs | 50 | 3.5 |
Comparison in Photocatalytic Methane Production/Conversion
Silver catalysts are pivotal in converting CO₂ to methane and methane to methanol under mild conditions.
Key Findings:
- Ag-HMO Single-Atom Catalyst : Achieves 6.0 µmol h⁻¹ mg⁻¹ CH₄ production from CO₂, surpassing Rh/Al₂O₃ (0.60 µmol h⁻¹ mg⁻¹) .
- Ag-Cu Dual-Atom Catalysts: Enhance methanol yield (12.3%) in direct methane oxidation, outperforming conventional Pt-based catalysts (8.1%) .
Table 3: Photocatalytic CO₂-to-CH₄ Conversion Efficiency
Catalyst | CH₄ Production Rate (µmol h⁻¹ mg⁻¹) | Light Source | Reference |
---|---|---|---|
Ag-HMO | 6.0 | Visible | |
Rh/Al₂O₃ | 0.60 | UV | |
In₂O₃-X(OH)y | 0.60 | Visible |
Data Tables Summarizing Key Findings
See Tables 1–3 above for consolidated performance metrics.
Biological Activity
The compound "methane;silver" refers to a combination of methane (a simple hydrocarbon) and silver, particularly in the context of silver nanoparticles (AgNPs). This article explores the biological activity associated with silver nanoparticles, particularly their antimicrobial, antioxidant, and cytotoxic properties, while considering the implications of methane as a potential carrier or medium for these nanoparticles.
Overview of Silver Nanoparticles
Silver nanoparticles have gained attention due to their unique properties, including high surface area-to-volume ratio and antimicrobial activity. These nanoparticles exhibit significant biological activities that make them suitable for various applications in medicine and environmental science.
Antimicrobial Activity
Silver nanoparticles are well-documented for their antimicrobial properties. They have been shown to be effective against a wide range of bacteria and fungi. The mechanism of action involves:
- Release of Ag+ Ions : Upon dissolution, silver nanoparticles release Ag+ ions, which can penetrate bacterial cell walls and disrupt cellular functions.
- Reactive Oxygen Species (ROS) Generation : The oxidative stress induced by ROS leads to cell membrane damage and ultimately cell death .
Case Study: Antibacterial Efficacy
A study evaluated the antibacterial activity of silver nanoparticles against Escherichia coli and Staphylococcus aureus. The results indicated that smaller-sized nanoparticles (10.8 nm) exhibited higher toxicity compared to larger ones (22.7 nm), demonstrating a direct relationship between particle size and antibacterial efficacy .
Nanoparticle Size (nm) | Minimum Inhibitory Concentration (MIC) | Toxicity Level |
---|---|---|
10.8 | 0.6 × 10⁻⁴ mol/L | High |
22.7 | 2.8 × 10⁻⁴ mol/L | Moderate |
Antioxidant Activity
Silver nanoparticles also possess antioxidant properties. They can scavenge free radicals, thereby reducing oxidative stress in cells. This activity is particularly beneficial in preventing cellular damage associated with various diseases.
- DPPH Assay : The antioxidant capacity of silver nanoparticles was assessed using the DPPH radical scavenging assay, which showed significant radical scavenging activity .
Cytotoxicity Studies
Cytotoxicity is a critical aspect when evaluating the safety of silver nanoparticles for biomedical applications. Studies have shown that while AgNPs can effectively kill cancer cells, they also exhibit cytotoxic effects on normal cells.
- MTT Assay : A study involving A-431 osteosarcoma cell lines demonstrated that AgNPs reduced cell viability in a dose-dependent manner, indicating potential therapeutic applications in cancer treatment .
Table: Cytotoxic Effects of Silver Nanoparticles on A-431 Cells
Concentration (μg/ml) | Cell Viability (%) |
---|---|
10 | 90 |
50 | 70 |
100 | 50 |
150 | 30 |
250 | 10 |
Implications of Methane as a Carrier
While silver nanoparticles are primarily studied for their direct biological activities, the role of methane as a carrier or medium is less explored. Methane could potentially influence the stability and distribution of silver nanoparticles in biological systems.
- Potential Applications : If methane can effectively stabilize silver nanoparticles in an aqueous environment, it may enhance their delivery to target sites, improving their efficacy as antimicrobial or therapeutic agents.
Properties
Molecular Formula |
C23H92Ag |
---|---|
Molecular Weight |
476.8 g/mol |
IUPAC Name |
methane;silver |
InChI |
InChI=1S/23CH4.Ag/h23*1H4; |
InChI Key |
VUXRQACBKWNROL-UHFFFAOYSA-N |
Canonical SMILES |
C.C.C.C.C.C.C.C.C.C.C.C.C.C.C.C.C.C.C.C.C.C.C.[Ag] |
Origin of Product |
United States |
Disclaimer and Information on In-Vitro Research Products
Please be aware that all articles and product information presented on BenchChem are intended solely for informational purposes. The products available for purchase on BenchChem are specifically designed for in-vitro studies, which are conducted outside of living organisms. In-vitro studies, derived from the Latin term "in glass," involve experiments performed in controlled laboratory settings using cells or tissues. It is important to note that these products are not categorized as medicines or drugs, and they have not received approval from the FDA for the prevention, treatment, or cure of any medical condition, ailment, or disease. We must emphasize that any form of bodily introduction of these products into humans or animals is strictly prohibited by law. It is essential to adhere to these guidelines to ensure compliance with legal and ethical standards in research and experimentation.