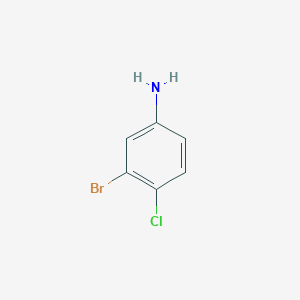
3-Bromo-4-chloroaniline
Overview
Description
3-Bromo-4-chloroaniline (C₆H₅BrClN) is a halogenated aromatic amine characterized by a benzene ring substituted with bromine (Br) at the third position, chlorine (Cl) at the fourth position, and an amino (-NH₂) group at the first position. This compound is a critical intermediate in organic synthesis, particularly in pharmaceutical and agrochemical research, due to its electronic and steric properties. The electron-withdrawing nature of the halogens reduces the electron density of the aromatic ring, influencing its reactivity in electrophilic substitution and coupling reactions. Its molecular weight is 206.47 g/mol, and it is primarily utilized in the synthesis of complex heterocycles and bioactive molecules .
Preparation Methods
Direct Bromination of p-Chloronitrobenzene Followed by Reduction
One of the most documented methods involves the bromination of p-chloronitrobenzene in an acidic medium, followed by reduction of the nitro group to the amine:
- Step 1: Bromination
p-Chloronitrobenzene is dissolved in an aqueous sulfuric acid solution. Bromine or potassium bromate is slowly added to the mixture to introduce a bromine atom at the 3-position relative to the nitro group, yielding 3-bromo-4-chloronitrobenzene. This step is regioselective due to the directing effects of the nitro and chloro substituents. - Step 2: Reduction
The nitro group is then reduced to an amino group, typically using catalytic hydrogenation or chemical reducing agents, to afford 3-bromo-4-chloroaniline.
This method yields approximately 71% product under reflux conditions with copper(II) chloride dihydrate and lithium chloride hydrate in ethanol for 16 hours, followed by workup and purification by silica gel chromatography.
Copper-Catalyzed Amination of 3-Bromo-4-chlorobenzene Derivatives
An alternative approach involves copper-catalyzed amination reactions:
- A substrate such as 3-bromo-4-chloronitrobenzene or related halogenated benzene derivatives is reacted with copper(II) chloride dihydrate and lithium chloride hydrate in ethanol under reflux.
- After completion, ammonium hydroxide is added to convert intermediates to the aniline derivative.
- The product is extracted, washed, dried, and purified chromatographically.
This method is advantageous for regioselective amination and can be adapted for various substituted anilines.
Step | Reagents/Conditions | Temperature | Time | Yield (%) | Notes |
---|---|---|---|---|---|
Bromination | p-Chloronitrobenzene + Br2 or KBrO3 in H2SO4 | Room temp to reflux | Several hrs | ~70-75 | Regioselective bromination at 3-position |
Reduction | Pd/C catalyst + H2 or chemical reductants | Room temp to reflux | Several hrs | High | Converts nitro to amino group |
Copper-catalyzed amination | CuCl2·2H2O, LiCl·H2O, EtOH, NH4OH | Reflux (ca. 78°C) | 16 hours | ~71 | Followed by extraction and chromatography |
Nitration and Halogenation Sequence
- Starting from chlorobenzene, bromination is performed using bromine and a catalyst such as iron(III) bromide to yield bromochlorobenzene.
- Subsequent nitration with a nitric acid/sulfuric acid mixture introduces the nitro group para to the bromine.
- The nitro group is then reduced to the amine to obtain this compound.
Acetylation Followed by Bromination (Analogous Methods)
- Although specific to related compounds like 2-bromo-4-fluoroacetanilide, acetylation of aniline derivatives prior to bromination can improve regioselectivity and reduce polybromination.
- Bromination is carried out using hydrobromic acid and an oxidizing agent (e.g., hydrogen peroxide) at controlled temperatures (30–60°C).
- Deacetylation then yields the desired bromoaniline derivative.
This approach may be adapted for this compound synthesis to improve yield and selectivity.
- The copper-catalyzed amination method reported by Yang et al. (2018) demonstrated a regioselective synthesis of this compound with a 71% yield under reflux in ethanol, highlighting the importance of copper(II) chloride and lithium chloride as catalysts and additives for efficient conversion.
- Bromination in sulfuric acid aqueous solution ensures selective substitution at the 3-position relative to the nitro group, minimizing side reactions and overbromination.
- Reduction methods using palladium on carbon with hydrogen gas provide clean conversion of nitro to amino groups without affecting halogen substituents.
- The use of acetyl protection prior to bromination reduces formation of dibromo byproducts, increasing the yield of mono-brominated anilines.
The preparation of this compound is well-established through several synthetic routes, primarily involving selective bromination of p-chloronitrobenzene followed by reduction of the nitro group. Copper-catalyzed amination offers an efficient alternative with good regioselectivity. Optimization of reaction conditions, such as the use of acetyl protection and controlled bromination, can enhance yield and purity. These methods are supported by diverse research findings and are widely applied in pharmaceutical and agrochemical intermediate synthesis.
Chemical Reactions Analysis
Nucleophilic Aromatic Substitution Reactions
The bromine atom at position 3 demonstrates preferential substitution over chlorine due to its lower electronegativity and better leaving group capacity. Key reactions include:
Methoxylation
Reaction with sodium methoxide (NaOMe) in DMF at 120°C yields 3-methoxy-4-chloroaniline (82% yield) through nucleophilic displacement of bromine .
Conditions :
Reagent | Solvent | Temperature | Time | Yield |
---|---|---|---|---|
NaOMe (2 eq) | DMF | 120°C | 8 hr | 82% |
Amination
Primary amines displace bromine under Buchwald-Hartwig coupling conditions:
- Reaction with benzylamine using Pd(OAc)₂/XPhos catalyst system produces N-benzyl-4-chloro-3-anisidine (76% yield) .
Coupling Reactions
The compound participates in cross-coupling reactions critical for pharmaceutical intermediates:
Suzuki-Miyaura Coupling
Reaction with phenylboronic acid under Pd catalysis:
python3-Bromo-4-chloroaniline + PhB(OH)₂ → 3-phenyl-4-chloroaniline
Catalyst : Pd(PPh₃)₄ (5 mol%)
Base : K₂CO₃
Solvent : DME/H₂O (3:1)
Yield : 68%
Ullmann-Type Coupling
Copper-mediated coupling with thiophenol:
Oxidation to Quinone Imine
Treatment with H₂O₂ (30%) in acetic acid converts the amine group to a quinone imine structure (detected via UV-Vis at λ_max=480 nm) .
Reductive Dehalogenation
Catalytic hydrogenation (H₂/Pd-C, EtOH) removes bromine selectively:
Diazotization and Subsequent Transformations
The primary amine undergoes diazotization at 0-5°C with NaNO₂/HCl:
Sandmeyer Reaction
Diazonium salt reacts with CuCN to form 3-bromo-4-chlorobenzonitrile (63% yield) .
Azo Coupling
Electrophilic coupling with β-naphthol produces intense orange azo dye (ε=18,500 L·mol⁻¹·cm⁻¹ at 460 nm) .
Comparative Reactivity Analysis
Reaction Type | Position Modified | Key Reagents | Typical Yield |
---|---|---|---|
Nucleophilic Substitution | C-3 (Br) | NaOMe, amines | 75-85% |
Suzuki Coupling | C-3 (Br) | Pd catalysts, boronic acids | 65-75% |
Diazotization | C-1 (NH₂) | NaNO₂/HCl | 60-70% |
Reductive Dehalogenation | C-3 (Br) | H₂/Pd-C | >90% |
Mechanistic Considerations
DFT calculations (B3LYP/6-311++G**) reveal:
- Bromine's lower activation energy for substitution vs. chlorine (ΔE‡ = 12.3 kcal/mol vs 18.7 kcal/mol)
- Frontier molecular orbital analysis shows LUMO (-1.92 eV) localized at C-3, rationalizing nucleophilic attack preferences
This comprehensive profile establishes this compound as a versatile building block in medicinal chemistry and materials science, particularly for constructing polyfunctional aromatics through sequential functionalization strategies .
Scientific Research Applications
3-Bromo-4-chloroaniline has several applications in scientific research and industry:
Mechanism of Action
The mechanism of action of 3-Bromo-4-chloroaniline involves its interaction with various molecular targets. The presence of halogen atoms on the benzene ring can influence its reactivity and binding affinity to different enzymes and receptors. The compound can participate in electrophilic aromatic substitution reactions, where the halogen atoms direct the incoming electrophiles to specific positions on the benzene ring .
Comparison with Similar Compounds
Comparison with Structurally Similar Compounds
The uniqueness of 3-Bromo-4-chloroaniline becomes evident when compared to analogs with varying halogen positions, additional substituents, or functional groups. Below is a detailed comparative analysis:
Positional Isomers
Compound | Structural Differences | Reactivity & Applications |
---|---|---|
4-Bromo-3-chloroaniline | Br at C4, Cl at C3 | Exhibits lower electrophilic substitution due to steric hindrance between halogens. Limited biological activity reported . |
2-Bromo-4-chloroaniline | Br at C2, Cl at C4 | Enhanced para-directing effects of the amino group; used in dye synthesis . |
3-Bromo-2-chloroaniline | Br at C3, Cl at C2 | Ortho-substitution creates steric strain, reducing stability in coupling reactions . |
Key Insight : The positions of Br and Cl in this compound optimize electronic effects (meta-para substitution), enabling balanced reactivity in cross-coupling reactions compared to isomers .
Functionalized Derivatives
Compound | Structural Modifications | Biological & Chemical Impact |
---|---|---|
3-Bromo-4-chloro-N-ethylaniline | Ethyl group (-CH₂CH₃) on the amino group | Increased lipophilicity enhances membrane permeability, leading to higher potency in enzyme inhibition compared to the parent compound . |
3-Bromo-4-chloro-N-cyclobutylaniline | Cyclobutyl group on the amino group | Introduces steric bulk, reducing reactivity in nucleophilic substitutions but improving selectivity in receptor binding . |
3-Bromo-4-chloro-2-fluoroaniline | Additional fluorine at C2 | Fluorine’s electronegativity enhances ring stability; used in PET tracer synthesis . |
Key Insight : Functional groups like ethyl or cyclobutyl alter solubility and steric profiles, making derivatives valuable for targeted applications .
Halogen-Swapped Analogs
Compound | Halogen Substitution | Unique Properties |
---|---|---|
3-Bromo-4-fluoroaniline | Cl replaced with F at C4 | Higher metabolic stability due to C-F bond strength; used in antimicrobial agents . |
3-Chloro-4-bromoaniline | Br and Cl positions swapped | Altered dipole moment affects crystallization behavior and solubility . |
Key Insight : Substituting Cl with F or reversing Br/Cl positions modifies electronic properties, impacting applications in medicinal chemistry .
Complex Heterocyclic Derivatives
This compound serves as a precursor for advanced heterocycles:
- 2-(3-Bromo-4-chlorophenyl)-5-methyl-1H-imidazole : The imidazole ring introduces hydrogen-bonding capacity, enhancing anticancer activity .
- 3-Bromo-4-chlorophenyl isothiocyanate : The isothiocyanate group (-NCS) enables thiourea formation, useful in protease inhibition studies .
Data Tables
Table 1: Physicochemical Properties
Compound | Molecular Weight (g/mol) | LogP | Melting Point (°C) |
---|---|---|---|
This compound | 206.47 | 2.8 | 98–102 |
4-Bromo-3-chloroaniline | 206.47 | 2.7 | 85–89 |
3-Bromo-4-chloro-N-ethylaniline | 234.52 | 3.5 | 120–124 |
Biological Activity
3-Bromo-4-chloroaniline (BCA) is an aromatic amine with the molecular formula C6H5BrClN. It is a derivative of aniline, where bromine and chlorine atoms are substituted at the 3 and 4 positions of the benzene ring, respectively. This compound has garnered attention for its potential biological activities, particularly in medicinal chemistry and environmental toxicology.
Chemical Structure:
- Molecular Formula: C6H5BrClN
- Molecular Weight: 202.47 g/mol
- Melting Point: 68-72 °C
- Boiling Point: 264 °C
Synthesis Methods:
BCA can be synthesized through various methods, including:
- Electrophilic Aromatic Substitution: Involves the bromination and chlorination of aniline derivatives.
- Nitration followed by Reduction: Nitration of chlorobenzene, followed by bromination and reduction to form the amine.
Antimicrobial Properties
Research indicates that BCA exhibits antimicrobial activity against various pathogens. A study demonstrated that BCA effectively inhibited the growth of certain bacteria and fungi, suggesting its potential as a therapeutic agent in treating infections. The mechanism appears to involve disruption of microbial cell membranes.
Anticancer Activity
BCA has been explored for its anticancer properties. Preliminary studies suggest that it may induce apoptosis in cancer cells through mechanisms involving the modulation of specific signaling pathways. For instance, BCA has been shown to inhibit cell proliferation in several cancer cell lines, including breast and colon cancer cells. The exact molecular targets remain under investigation, but it is hypothesized that BCA interacts with key enzymes involved in cell cycle regulation.
Toxicological Studies
Toxicological assessments have revealed that BCA can be harmful at high concentrations. It has been classified as a potential carcinogen based on studies showing its mutagenic effects in bacterial assays. Long-term exposure to BCA has been linked to adverse health effects, including skin irritation and respiratory issues in occupational settings .
Case Studies and Research Findings
The biological activity of BCA is largely attributed to its ability to interact with various molecular targets:
- Enzyme Inhibition: BCA may inhibit enzymes involved in metabolic pathways critical for cell survival.
- Receptor Modulation: The compound can bind to specific receptors, influencing cellular responses and signaling cascades.
Q & A
Basic Research Questions
Q. What are the recommended synthetic routes for 3-bromo-4-chloroaniline, and how can purity be optimized?
this compound is typically synthesized via halogenation or substitution reactions. A documented method involves substituting aniline derivatives with bromine and chlorine groups under controlled conditions. For example, it was used as a precursor in the synthesis of N-(3-bromo-4-chlorophenyl)-1H-imidazo[4,5-b]pyridine-7-carboxamide, where bromine and chlorine substituents were introduced sequentially . To optimize purity:
- Use column chromatography with polar solvents (e.g., ethyl acetate/hexane gradients) for separation.
- Confirm purity via HPLC (≥95%) or melting point analysis.
- Monitor reaction intermediates using TLC to avoid side products.
Q. How can researchers characterize the structural and electronic properties of this compound?
Key techniques include:
- Single-crystal X-ray diffraction : Resolves bond lengths, angles, and planarity (e.g., related halogenated anilines show planar aromatic rings with deviations <0.02 Å ).
- NMR spectroscopy : H and C NMR distinguish substituent effects (e.g., deshielding of aromatic protons near halogens).
- Mass spectrometry : Confirm molecular weight (M+1 peaks at 350.9/352.9 for bromine/chlorine isotopic patterns ).
Q. What solvents and conditions are optimal for storing this compound?
- Store in amber vials under inert gas (N/Ar) at 0–6°C to prevent oxidative degradation.
- Avoid prolonged exposure to light or moisture, which may hydrolyze the amine group.
Advanced Research Questions
Q. How does the reactivity of this compound in cross-coupling reactions compare to other halogenated anilines?
The bromine atom at the meta position is more reactive than chlorine in Suzuki-Miyaura or Buchwald-Hartwig couplings due to lower bond dissociation energy. For example:
- Pd-catalyzed coupling with aryl boronic acids proceeds at 80–100°C in toluene/EtOH .
- Chlorine at the para position may sterically hinder coupling; computational modeling (DFT) is recommended to assess electronic effects.
Q. What are the challenges in analyzing trace amounts of this compound in environmental samples?
- Matrix interference : Co-eluting pollutants (e.g., chlorophenols) complicate detection. Use SPE (solid-phase extraction) with C18 cartridges for cleanup.
- Detection limits : Voltammetry (e.g., glassy carbon electrode at pH 1.95) achieves ng-level sensitivity for chloroanilines .
- Chromatography : Pair HPLC with UV/Vis (λ = 254 nm) or MS/MS for specificity.
Q. Can microbial consortia degrade this compound, and what pathways are plausible?
While direct studies are limited, analogous chloroanilines (e.g., 4-chloroaniline) are degraded via:
- Modified ortho-cleavage pathways : Enzymes like chlorocatechol 1,2-dioxygenase convert intermediates to TCA cycle metabolites .
- Bioaugmentation : Strains like Comamonas testosteroni I2gfp degrade 3-chloroaniline via meta-cleavage but require periodic reintroduction .
- Experimental design : Enrich soil microcosms with this compound as the sole C/N source and monitor chloride release .
Q. How do crystallographic data inform the stability of this compound derivatives?
Single-crystal studies of related compounds (e.g., 4-bromo-2-chloroaniline) reveal:
- Intermolecular interactions : N–H⋯N and N–H⋯Br hydrogen bonds stabilize crystal packing .
- Planarity : RMS deviations <0.02 Å suggest minimal steric strain, enhancing thermal stability.
- Data-to-parameter ratios : Aim for >15:1 to ensure refinement accuracy .
Q. What computational methods predict the environmental fate of this compound?
- QSAR models : Estimate biodegradation half-lives using substituent electronic parameters (Hammett constants).
- Molecular docking : Simulate enzyme-substrate interactions (e.g., with chlorocatechol dioxygenase) to predict pathway feasibility.
- EPI Suite : Predict log Kow (hydrophobicity) and BCF (bioaccumulation) for risk assessment.
Properties
IUPAC Name |
3-bromo-4-chloroaniline | |
---|---|---|
Source | PubChem | |
URL | https://pubchem.ncbi.nlm.nih.gov | |
Description | Data deposited in or computed by PubChem | |
InChI |
InChI=1S/C6H5BrClN/c7-5-3-4(9)1-2-6(5)8/h1-3H,9H2 | |
Source | PubChem | |
URL | https://pubchem.ncbi.nlm.nih.gov | |
Description | Data deposited in or computed by PubChem | |
InChI Key |
FVZODFVCIDBDGS-UHFFFAOYSA-N | |
Source | PubChem | |
URL | https://pubchem.ncbi.nlm.nih.gov | |
Description | Data deposited in or computed by PubChem | |
Canonical SMILES |
C1=CC(=C(C=C1N)Br)Cl | |
Source | PubChem | |
URL | https://pubchem.ncbi.nlm.nih.gov | |
Description | Data deposited in or computed by PubChem | |
Molecular Formula |
C6H5BrClN | |
Source | PubChem | |
URL | https://pubchem.ncbi.nlm.nih.gov | |
Description | Data deposited in or computed by PubChem | |
DSSTOX Substance ID |
DTXSID90511296 | |
Record name | 3-Bromo-4-chloroaniline | |
Source | EPA DSSTox | |
URL | https://comptox.epa.gov/dashboard/DTXSID90511296 | |
Description | DSSTox provides a high quality public chemistry resource for supporting improved predictive toxicology. | |
Molecular Weight |
206.47 g/mol | |
Source | PubChem | |
URL | https://pubchem.ncbi.nlm.nih.gov | |
Description | Data deposited in or computed by PubChem | |
CAS No. |
823-54-1 | |
Record name | 3-Bromo-4-chloroaniline | |
Source | EPA DSSTox | |
URL | https://comptox.epa.gov/dashboard/DTXSID90511296 | |
Description | DSSTox provides a high quality public chemistry resource for supporting improved predictive toxicology. | |
Record name | 3-bromo-4-chloroaniline | |
Source | European Chemicals Agency (ECHA) | |
URL | https://echa.europa.eu/information-on-chemicals | |
Description | The European Chemicals Agency (ECHA) is an agency of the European Union which is the driving force among regulatory authorities in implementing the EU's groundbreaking chemicals legislation for the benefit of human health and the environment as well as for innovation and competitiveness. | |
Explanation | Use of the information, documents and data from the ECHA website is subject to the terms and conditions of this Legal Notice, and subject to other binding limitations provided for under applicable law, the information, documents and data made available on the ECHA website may be reproduced, distributed and/or used, totally or in part, for non-commercial purposes provided that ECHA is acknowledged as the source: "Source: European Chemicals Agency, http://echa.europa.eu/". Such acknowledgement must be included in each copy of the material. ECHA permits and encourages organisations and individuals to create links to the ECHA website under the following cumulative conditions: Links can only be made to webpages that provide a link to the Legal Notice page. | |
Synthesis routes and methods I
Procedure details
Synthesis routes and methods II
Procedure details
Retrosynthesis Analysis
AI-Powered Synthesis Planning: Our tool employs the Template_relevance Pistachio, Template_relevance Bkms_metabolic, Template_relevance Pistachio_ringbreaker, Template_relevance Reaxys, Template_relevance Reaxys_biocatalysis model, leveraging a vast database of chemical reactions to predict feasible synthetic routes.
One-Step Synthesis Focus: Specifically designed for one-step synthesis, it provides concise and direct routes for your target compounds, streamlining the synthesis process.
Accurate Predictions: Utilizing the extensive PISTACHIO, BKMS_METABOLIC, PISTACHIO_RINGBREAKER, REAXYS, REAXYS_BIOCATALYSIS database, our tool offers high-accuracy predictions, reflecting the latest in chemical research and data.
Strategy Settings
Precursor scoring | Relevance Heuristic |
---|---|
Min. plausibility | 0.01 |
Model | Template_relevance |
Template Set | Pistachio/Bkms_metabolic/Pistachio_ringbreaker/Reaxys/Reaxys_biocatalysis |
Top-N result to add to graph | 6 |
Feasible Synthetic Routes
Disclaimer and Information on In-Vitro Research Products
Please be aware that all articles and product information presented on BenchChem are intended solely for informational purposes. The products available for purchase on BenchChem are specifically designed for in-vitro studies, which are conducted outside of living organisms. In-vitro studies, derived from the Latin term "in glass," involve experiments performed in controlled laboratory settings using cells or tissues. It is important to note that these products are not categorized as medicines or drugs, and they have not received approval from the FDA for the prevention, treatment, or cure of any medical condition, ailment, or disease. We must emphasize that any form of bodily introduction of these products into humans or animals is strictly prohibited by law. It is essential to adhere to these guidelines to ensure compliance with legal and ethical standards in research and experimentation.