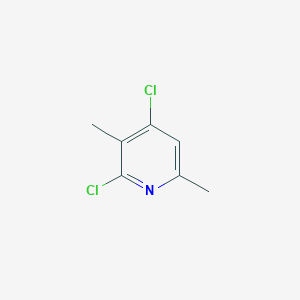
2,4-Dichloro-3,6-dimethylpyridine
Overview
Description
2,4-Dichloro-3,6-dimethylpyridine is an organic compound with the molecular formula C7H7Cl2N. It is a derivative of pyridine, characterized by the presence of two chlorine atoms at the 2nd and 4th positions and two methyl groups at the 3rd and 6th positions on the pyridine ring. This compound is known for its applications in various fields, including organic synthesis, pharmaceuticals, and agrochemicals .
Preparation Methods
Synthetic Routes and Reaction Conditions: 2,4-Dichloro-3,6-dimethylpyridine can be synthesized through several methods. One common approach involves the chlorination of 3,6-dimethylpyridine using chlorine gas in the presence of a catalyst such as iron(III) chloride. The reaction typically occurs at elevated temperatures to ensure complete chlorination .
Industrial Production Methods: In industrial settings, the production of this compound often involves large-scale chlorination processes. The reaction conditions are optimized to maximize yield and purity, with careful control of temperature, pressure, and reactant concentrations. The product is then purified through distillation or recrystallization .
Chemical Reactions Analysis
Types of Reactions: 2,4-Dichloro-3,6-dimethylpyridine undergoes various chemical reactions, including:
Substitution Reactions: The chlorine atoms can be substituted by other nucleophiles such as amines, thiols, or alkoxides under appropriate conditions.
Oxidation Reactions: The methyl groups can be oxidized to form corresponding carboxylic acids or aldehydes.
Reduction Reactions: The compound can be reduced to form 2,4-dichloro-3,6-dimethylpiperidine.
Common Reagents and Conditions:
Substitution Reactions: Reagents like sodium amide or potassium tert-butoxide in solvents such as dimethyl sulfoxide (DMSO) or tetrahydrofuran (THF).
Oxidation Reactions: Oxidizing agents like potassium permanganate or chromium trioxide in acidic or basic media.
Reduction Reactions: Reducing agents such as lithium aluminum hydride or sodium borohydride in anhydrous solvents.
Major Products:
- Substitution reactions yield various substituted pyridines.
- Oxidation reactions produce carboxylic acids or aldehydes.
- Reduction reactions result in the formation of piperidine derivatives .
Scientific Research Applications
2,4-Dichloro-3,6-dimethylpyridine is utilized in several scientific research applications:
Chemistry: It serves as an intermediate in the synthesis of complex organic molecules, including pharmaceuticals and agrochemicals.
Biology: The compound is used in the study of enzyme inhibition and receptor binding due to its structural similarity to biologically active pyridine derivatives.
Medicine: It is investigated for its potential therapeutic properties, including antimicrobial and anticancer activities.
Industry: The compound is employed in the production of pesticides, herbicides, and other agrochemicals.
Mechanism of Action
The mechanism of action of 2,4-dichloro-3,6-dimethylpyridine involves its interaction with specific molecular targets. The compound can inhibit certain enzymes by binding to their active sites, thereby blocking substrate access. Additionally, it can interact with cellular receptors, modulating signal transduction pathways. These interactions result in various biological effects, including antimicrobial and anticancer activities .
Comparison with Similar Compounds
2,4-Dichloro-6-methylpyridine: Similar structure but with one less methyl group.
2,6-Dichloro-3-methylpyridine: Similar structure but with chlorine atoms at different positions.
2-Chloro-4,6-dimethylpyridine: Similar structure but with one less chlorine atom.
Uniqueness: 2,4-Dichloro-3,6-dimethylpyridine is unique due to its specific substitution pattern, which imparts distinct chemical and biological properties. The presence of both chlorine and methyl groups at specific positions on the pyridine ring enhances its reactivity and potential for diverse applications .
Biological Activity
2,4-Dichloro-3,6-dimethylpyridine is a compound of significant interest in medicinal chemistry due to its diverse biological activities. This article reviews the compound's synthesis, biological effects, and potential applications in various therapeutic areas.
This compound is a chlorinated derivative of pyridine, characterized by the presence of two chlorine atoms at positions 2 and 4 and methyl groups at positions 3 and 6. Its chemical formula is C8H8Cl2N. The synthesis typically involves nucleophilic substitution reactions on pyridine derivatives, which can yield various regioisomers depending on the reaction conditions used .
Anticancer Properties
Recent studies have highlighted the anticancer potential of this compound. It exhibits cytotoxic effects against several cancer cell lines. For instance, a study demonstrated that derivatives containing similar structural motifs showed significant activity against melanoma cells (A375) and breast cancer cells (MCF-7) using the MTT assay. The compound induced apoptosis in these cells in a dose-dependent manner .
Table 1: Cytotoxic Activity Against Cancer Cell Lines
Cell Line | IC50 (µM) | Mechanism of Action |
---|---|---|
A375 (Melanoma) | 100 | Induction of apoptosis |
MCF-7 (Breast) | 150 | Inhibition of cell proliferation |
HepG2 (Liver) | 80 | Disruption of mitochondrial function |
Antibacterial Activity
In addition to its anticancer properties, this compound has shown promising antibacterial activity. Research indicates that it can inhibit the growth of both Gram-positive and Gram-negative bacteria. The mechanism appears to involve disruption of bacterial cell wall synthesis and interference with bacterial metabolic pathways .
Case Study: Antibacterial Efficacy
A study evaluated the antibacterial activity of this compound against Staphylococcus aureus and Escherichia coli. The compound demonstrated a minimum inhibitory concentration (MIC) of 32 µg/mL against S. aureus and 64 µg/mL against E. coli.
The biological activity of this compound is attributed to several mechanisms:
- Apoptosis Induction : The compound triggers apoptotic pathways in cancer cells by activating caspases and promoting DNA fragmentation.
- Antibacterial Mechanisms : It disrupts bacterial cell wall integrity and inhibits key enzymes involved in bacterial metabolism.
- Molecular Docking Studies : Computational studies suggest that the compound interacts with various biological targets through non-covalent interactions, enhancing its therapeutic potential .
Properties
IUPAC Name |
2,4-dichloro-3,6-dimethylpyridine | |
---|---|---|
Source | PubChem | |
URL | https://pubchem.ncbi.nlm.nih.gov | |
Description | Data deposited in or computed by PubChem | |
InChI |
InChI=1S/C7H7Cl2N/c1-4-3-6(8)5(2)7(9)10-4/h3H,1-2H3 | |
Source | PubChem | |
URL | https://pubchem.ncbi.nlm.nih.gov | |
Description | Data deposited in or computed by PubChem | |
InChI Key |
YGUQXFNMLHUBNR-UHFFFAOYSA-N | |
Source | PubChem | |
URL | https://pubchem.ncbi.nlm.nih.gov | |
Description | Data deposited in or computed by PubChem | |
Canonical SMILES |
CC1=CC(=C(C(=N1)Cl)C)Cl | |
Source | PubChem | |
URL | https://pubchem.ncbi.nlm.nih.gov | |
Description | Data deposited in or computed by PubChem | |
Molecular Formula |
C7H7Cl2N | |
Source | PubChem | |
URL | https://pubchem.ncbi.nlm.nih.gov | |
Description | Data deposited in or computed by PubChem | |
DSSTOX Substance ID |
DTXSID90454598 | |
Record name | 2,4-Dichloro-3,6-dimethylpyridine | |
Source | EPA DSSTox | |
URL | https://comptox.epa.gov/dashboard/DTXSID90454598 | |
Description | DSSTox provides a high quality public chemistry resource for supporting improved predictive toxicology. | |
Molecular Weight |
176.04 g/mol | |
Source | PubChem | |
URL | https://pubchem.ncbi.nlm.nih.gov | |
Description | Data deposited in or computed by PubChem | |
CAS No. |
83791-90-6 | |
Record name | 2,4-Dichloro-3,6-dimethylpyridine | |
Source | EPA DSSTox | |
URL | https://comptox.epa.gov/dashboard/DTXSID90454598 | |
Description | DSSTox provides a high quality public chemistry resource for supporting improved predictive toxicology. | |
Synthesis routes and methods I
Procedure details
Synthesis routes and methods II
Procedure details
Retrosynthesis Analysis
AI-Powered Synthesis Planning: Our tool employs the Template_relevance Pistachio, Template_relevance Bkms_metabolic, Template_relevance Pistachio_ringbreaker, Template_relevance Reaxys, Template_relevance Reaxys_biocatalysis model, leveraging a vast database of chemical reactions to predict feasible synthetic routes.
One-Step Synthesis Focus: Specifically designed for one-step synthesis, it provides concise and direct routes for your target compounds, streamlining the synthesis process.
Accurate Predictions: Utilizing the extensive PISTACHIO, BKMS_METABOLIC, PISTACHIO_RINGBREAKER, REAXYS, REAXYS_BIOCATALYSIS database, our tool offers high-accuracy predictions, reflecting the latest in chemical research and data.
Strategy Settings
Precursor scoring | Relevance Heuristic |
---|---|
Min. plausibility | 0.01 |
Model | Template_relevance |
Template Set | Pistachio/Bkms_metabolic/Pistachio_ringbreaker/Reaxys/Reaxys_biocatalysis |
Top-N result to add to graph | 6 |
Feasible Synthetic Routes
Disclaimer and Information on In-Vitro Research Products
Please be aware that all articles and product information presented on BenchChem are intended solely for informational purposes. The products available for purchase on BenchChem are specifically designed for in-vitro studies, which are conducted outside of living organisms. In-vitro studies, derived from the Latin term "in glass," involve experiments performed in controlled laboratory settings using cells or tissues. It is important to note that these products are not categorized as medicines or drugs, and they have not received approval from the FDA for the prevention, treatment, or cure of any medical condition, ailment, or disease. We must emphasize that any form of bodily introduction of these products into humans or animals is strictly prohibited by law. It is essential to adhere to these guidelines to ensure compliance with legal and ethical standards in research and experimentation.