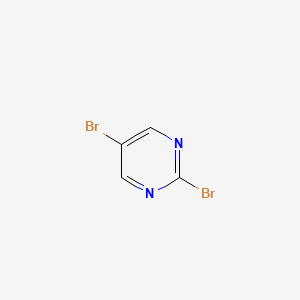
2,5-Dibromopyrimidine
Overview
Description
2,5-Dibromopyrimidine is an organic compound with the molecular formula C4H2Br2N2 It is a derivative of pyrimidine, where two bromine atoms are substituted at the 2nd and 5th positions of the pyrimidine ring
Preparation Methods
Synthetic Routes and Reaction Conditions
2,5-Dibromopyrimidine can be synthesized through several methods. One common approach involves the bromination of pyrimidine derivatives. For instance, 5-bromo-2-chloropyrimidine can be reacted with a solution of hydrogen bromide in a non-aqueous acid at temperatures ranging from 0°C to 120°C . This reaction yields this compound with high efficiency.
Industrial Production Methods
In industrial settings, the production of this compound often involves similar bromination reactions but on a larger scale. The reaction conditions are optimized to ensure high yield and purity of the product. The crude product obtained from the reaction mixture can be purified using standard methods such as recrystallization, distillation, or chromatographic techniques .
Chemical Reactions Analysis
Types of Reactions
2,5-Dibromopyrimidine undergoes various types of chemical reactions, including:
Substitution Reactions: The bromine atoms can be substituted with other nucleophiles, such as amines or thiols, under appropriate conditions.
Coupling Reactions: It can participate in cross-coupling reactions, such as Suzuki or Stille coupling, to form carbon-carbon bonds.
Common Reagents and Conditions
Substitution Reactions: Common reagents include nucleophiles like amines, thiols, or alkoxides. The reactions are typically carried out in polar solvents such as dimethylformamide or dimethyl sulfoxide at elevated temperatures.
Coupling Reactions: Palladium catalysts, such as tetrakis(triphenylphosphine)palladium(0), are often used in the presence of bases like potassium carbonate or cesium carbonate.
Major Products Formed
Substitution Reactions: Products include various substituted pyrimidines, depending on the nucleophile used.
Coupling Reactions: Products are typically biaryl compounds or other complex organic molecules.
Scientific Research Applications
2,5-Dibromopyrimidine has a wide range of applications in scientific research:
Chemistry: It is used as a building block in the synthesis of more complex organic molecules, including pharmaceuticals and agrochemicals.
Biology: It serves as a precursor for the synthesis of biologically active compounds that can be used in drug discovery and development.
Medicine: Derivatives of this compound are investigated for their potential therapeutic properties, including antiviral and anticancer activities.
Industry: It is used in the production of liquid crystals and other advanced materials.
Mechanism of Action
The mechanism of action of 2,5-dibromopyrimidine and its derivatives depends on the specific application and target. In medicinal chemistry, these compounds often interact with biological macromolecules, such as enzymes or receptors, to exert their effects. The bromine atoms can enhance the binding affinity of the compound to its target by forming halogen bonds or through hydrophobic interactions.
Comparison with Similar Compounds
Similar Compounds
- 2,4-Dibromopyrimidine
- 2,6-Dibromopyrimidine
- 2,5-Dibromopyridine
Comparison
2,5-Dibromopyrimidine is unique due to the specific positioning of the bromine atoms, which influences its reactivity and the types of reactions it can undergo. For example, 2,4-dibromopyrimidine and 2,6-dibromopyrimidine have different substitution patterns, leading to variations in their chemical behavior and applications. Similarly, 2,5-dibromopyridine, although structurally similar, has different electronic properties due to the presence of a nitrogen atom in the ring .
Properties
IUPAC Name |
2,5-dibromopyrimidine | |
---|---|---|
Source | PubChem | |
URL | https://pubchem.ncbi.nlm.nih.gov | |
Description | Data deposited in or computed by PubChem | |
InChI |
InChI=1S/C4H2Br2N2/c5-3-1-7-4(6)8-2-3/h1-2H | |
Source | PubChem | |
URL | https://pubchem.ncbi.nlm.nih.gov | |
Description | Data deposited in or computed by PubChem | |
InChI Key |
XAHITOJPIWZJHD-UHFFFAOYSA-N | |
Source | PubChem | |
URL | https://pubchem.ncbi.nlm.nih.gov | |
Description | Data deposited in or computed by PubChem | |
Canonical SMILES |
C1=C(C=NC(=N1)Br)Br | |
Source | PubChem | |
URL | https://pubchem.ncbi.nlm.nih.gov | |
Description | Data deposited in or computed by PubChem | |
Molecular Formula |
C4H2Br2N2 | |
Source | PubChem | |
URL | https://pubchem.ncbi.nlm.nih.gov | |
Description | Data deposited in or computed by PubChem | |
DSSTOX Substance ID |
DTXSID30449726 | |
Record name | 2,5-dibromopyrimidine | |
Source | EPA DSSTox | |
URL | https://comptox.epa.gov/dashboard/DTXSID30449726 | |
Description | DSSTox provides a high quality public chemistry resource for supporting improved predictive toxicology. | |
Molecular Weight |
237.88 g/mol | |
Source | PubChem | |
URL | https://pubchem.ncbi.nlm.nih.gov | |
Description | Data deposited in or computed by PubChem | |
CAS No. |
32779-37-6 | |
Record name | 2,5-dibromopyrimidine | |
Source | EPA DSSTox | |
URL | https://comptox.epa.gov/dashboard/DTXSID30449726 | |
Description | DSSTox provides a high quality public chemistry resource for supporting improved predictive toxicology. | |
Record name | 2,5-Dibromopyrimidine | |
Source | European Chemicals Agency (ECHA) | |
URL | https://echa.europa.eu/information-on-chemicals | |
Description | The European Chemicals Agency (ECHA) is an agency of the European Union which is the driving force among regulatory authorities in implementing the EU's groundbreaking chemicals legislation for the benefit of human health and the environment as well as for innovation and competitiveness. | |
Explanation | Use of the information, documents and data from the ECHA website is subject to the terms and conditions of this Legal Notice, and subject to other binding limitations provided for under applicable law, the information, documents and data made available on the ECHA website may be reproduced, distributed and/or used, totally or in part, for non-commercial purposes provided that ECHA is acknowledged as the source: "Source: European Chemicals Agency, http://echa.europa.eu/". Such acknowledgement must be included in each copy of the material. ECHA permits and encourages organisations and individuals to create links to the ECHA website under the following cumulative conditions: Links can only be made to webpages that provide a link to the Legal Notice page. | |
Retrosynthesis Analysis
AI-Powered Synthesis Planning: Our tool employs the Template_relevance Pistachio, Template_relevance Bkms_metabolic, Template_relevance Pistachio_ringbreaker, Template_relevance Reaxys, Template_relevance Reaxys_biocatalysis model, leveraging a vast database of chemical reactions to predict feasible synthetic routes.
One-Step Synthesis Focus: Specifically designed for one-step synthesis, it provides concise and direct routes for your target compounds, streamlining the synthesis process.
Accurate Predictions: Utilizing the extensive PISTACHIO, BKMS_METABOLIC, PISTACHIO_RINGBREAKER, REAXYS, REAXYS_BIOCATALYSIS database, our tool offers high-accuracy predictions, reflecting the latest in chemical research and data.
Strategy Settings
Precursor scoring | Relevance Heuristic |
---|---|
Min. plausibility | 0.01 |
Model | Template_relevance |
Template Set | Pistachio/Bkms_metabolic/Pistachio_ringbreaker/Reaxys/Reaxys_biocatalysis |
Top-N result to add to graph | 6 |
Feasible Synthetic Routes
Q1: What makes 2,5-dibromopyrimidine a valuable building block in organic synthesis?
A1: this compound serves as a versatile reagent in various palladium-catalyzed coupling reactions, such as the Sonogashira and Suzuki reactions. [, , ] Its two bromine atoms can be independently substituted with a wide range of aryl or heteroaryl groups, enabling the construction of diverse π-conjugated systems. [, , ] This versatility makes it particularly useful for developing novel polymers, oligomers, and conjugated porous polymers with tailored properties. [, , ]
Q2: How does this compound contribute to the development of high-performance X-ray detectors?
A2: While this compound itself is not directly incorporated into the X-ray detector, it plays a crucial role in synthesizing the active material. The research highlights the use of a derivative, this compound (DBPM), as a defect passivator in quasi-two-dimensional Ruddlesden-Popper (RP) perovskite materials for X-ray detection. [] DBPM effectively passivates Lewis acid defects within the RP perovskite structure, leading to enhanced performance. []
Q3: What are the benefits of using DBPM as a defect passivator in RP perovskite X-ray detectors?
A3: DBPM passivation in RP perovskite X-ray detectors brings about several advantages:
- Reduced ion migration: DBPM effectively suppresses ion migration within the perovskite, improving stability. []
- Improved optoelectronic properties: The passivation leads to enhanced charge transport and reduced trap-assisted recombination, resulting in higher sensitivity. []
- Enhanced operational stability: DBPM increases the activation energy of the RP perovskite, making the device more resistant to degradation under operational conditions. []
Q4: What is the significance of the electrochemical properties of poly(pyrimidine-2,5-diyl), a polymer derived from this compound?
A4: Poly(pyrimidine-2,5-diyl), synthesized from this compound, demonstrates interesting electrochemical behavior. [] It can be readily reduced both chemically and electrochemically, indicating its potential as an electron-accepting material. [] Notably, its electrochemical reduction occurs at a remarkably high potential, surpassing many other reported π-conjugated polymers. [] This property makes it a promising candidate for applications in organic electronics, particularly as an electron-transporting or n-type material.
Disclaimer and Information on In-Vitro Research Products
Please be aware that all articles and product information presented on BenchChem are intended solely for informational purposes. The products available for purchase on BenchChem are specifically designed for in-vitro studies, which are conducted outside of living organisms. In-vitro studies, derived from the Latin term "in glass," involve experiments performed in controlled laboratory settings using cells or tissues. It is important to note that these products are not categorized as medicines or drugs, and they have not received approval from the FDA for the prevention, treatment, or cure of any medical condition, ailment, or disease. We must emphasize that any form of bodily introduction of these products into humans or animals is strictly prohibited by law. It is essential to adhere to these guidelines to ensure compliance with legal and ethical standards in research and experimentation.