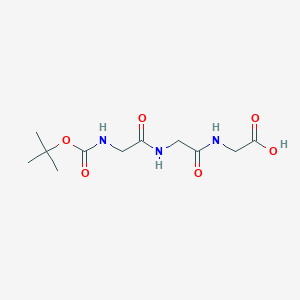
Boc-gly-gly-gly-OH
Overview
Description
N-tert-Butoxycarbonyl-glycyl-glycyl-glycine: is a tripeptide derivative where the amino group of the first glycine is protected by a tert-butoxycarbonyl group. This compound is commonly used in peptide synthesis due to its stability and ease of removal under acidic conditions.
Mechanism of Action
Target of Action
Boc-Gly-Gly-Gly-OH, also known as N-tert-butyloxycarbonyl-glycyl-glycine, is primarily used as a protective group for amino acids in peptide synthesis . It protects the amino group of glycine from side reactions during chemical synthesis .
Mode of Action
The compound acts as a protective group by reacting with the amino group of glycine, forming a stable compound that can withstand various chemical reactions . This protection is temporary and can be removed under appropriate conditions, allowing the peptide chain to extend .
Biochemical Pathways
The primary biochemical pathway involved in the action of this compound is peptide synthesis. During this process, the compound is added as a protective group to prevent the amino group of glycine from participating in unwanted side reactions . Once the desired reactions have taken place, the protective group is removed, allowing the peptide chain to continue its extension .
Result of Action
The use of this compound in peptide synthesis results in the successful elongation of peptide chains without interference from side reactions . By protecting the amino group of glycine, it ensures the integrity of the peptide chain and the accuracy of the synthesis process .
Action Environment
The action of this compound is influenced by the conditions of the chemical reaction it is involved in. It is stable under normal temperatures but may degrade under high temperatures, direct sunlight, or humid environments . During peptide synthesis, the reaction conditions must be carefully controlled to ensure the successful addition and removal of the protective group .
Preparation Methods
Synthetic Routes and Reaction Conditions: The synthesis of N-tert-Butoxycarbonyl-glycyl-glycyl-glycine typically involves the stepwise addition of glycine residues to a protected glycine starting material. The tert-butoxycarbonyl group is introduced using di-tert-butyl dicarbonate in the presence of a base such as sodium hydroxide or 4-dimethylaminopyridine in acetonitrile . The coupling of glycine residues can be achieved using standard peptide coupling reagents like N,N’-dicyclohexylcarbodiimide and 1-hydroxybenzotriazole .
Industrial Production Methods: In industrial settings, the production of N-tert-Butoxycarbonyl-glycyl-glycyl-glycine follows similar synthetic routes but on a larger scale. The use of automated peptide synthesizers and continuous flow reactors can enhance the efficiency and yield of the synthesis process .
Chemical Reactions Analysis
Types of Reactions: N-tert-Butoxycarbonyl-glycyl-glycyl-glycine undergoes various chemical reactions, including:
Deprotection: The tert-butoxycarbonyl group can be removed under acidic conditions using trifluoroacetic acid or hydrochloric acid in methanol.
Coupling Reactions: It can participate in peptide bond formation with other amino acids or peptides using coupling reagents like N,N’-dicyclohexylcarbodiimide.
Common Reagents and Conditions:
Deprotection: Trifluoroacetic acid in dichloromethane or hydrochloric acid in methanol.
Coupling: N,N’-dicyclohexylcarbodiimide and 1-hydroxybenzotriazole in anhydrous conditions.
Major Products Formed:
Deprotection: Glycyl-glycyl-glycine.
Coupling: Extended peptides or polypeptides depending on the reactants used.
Scientific Research Applications
Chemistry: N-tert-Butoxycarbonyl-glycyl-glycyl-glycine is widely used in peptide synthesis as a building block for more complex peptides and proteins .
Biology: In biological research, it serves as a model compound for studying peptide interactions and enzymatic processes .
Medicine: It is used in the development of peptide-based drugs and therapeutic agents .
Industry: In the pharmaceutical industry, it is employed in the synthesis of peptide drugs and as a precursor for various bioactive compounds .
Comparison with Similar Compounds
- N-tert-Butoxycarbonyl-glycine
- N-tert-Butoxycarbonyl-glycyl-glycine
- N-tert-Butoxycarbonyl-glycyl-glycyl-glycine
Uniqueness: N-tert-Butoxycarbonyl-glycyl-glycyl-glycine is unique due to its tripeptide structure, which provides more versatility in peptide synthesis compared to shorter Boc-protected peptides. Its stability and ease of deprotection make it a valuable compound in both research and industrial applications .
Properties
IUPAC Name |
2-[[2-[[2-[(2-methylpropan-2-yl)oxycarbonylamino]acetyl]amino]acetyl]amino]acetic acid | |
---|---|---|
Source | PubChem | |
URL | https://pubchem.ncbi.nlm.nih.gov | |
Description | Data deposited in or computed by PubChem | |
InChI |
InChI=1S/C11H19N3O6/c1-11(2,3)20-10(19)14-5-8(16)12-4-7(15)13-6-9(17)18/h4-6H2,1-3H3,(H,12,16)(H,13,15)(H,14,19)(H,17,18) | |
Source | PubChem | |
URL | https://pubchem.ncbi.nlm.nih.gov | |
Description | Data deposited in or computed by PubChem | |
InChI Key |
GHONIQQBOSTHSL-UHFFFAOYSA-N | |
Source | PubChem | |
URL | https://pubchem.ncbi.nlm.nih.gov | |
Description | Data deposited in or computed by PubChem | |
Canonical SMILES |
CC(C)(C)OC(=O)NCC(=O)NCC(=O)NCC(=O)O | |
Source | PubChem | |
URL | https://pubchem.ncbi.nlm.nih.gov | |
Description | Data deposited in or computed by PubChem | |
Molecular Formula |
C11H19N3O6 | |
Source | PubChem | |
URL | https://pubchem.ncbi.nlm.nih.gov | |
Description | Data deposited in or computed by PubChem | |
DSSTOX Substance ID |
DTXSID20427058 | |
Record name | BOC-GLY-GLY-GLY-OH | |
Source | EPA DSSTox | |
URL | https://comptox.epa.gov/dashboard/DTXSID20427058 | |
Description | DSSTox provides a high quality public chemistry resource for supporting improved predictive toxicology. | |
Molecular Weight |
289.29 g/mol | |
Source | PubChem | |
URL | https://pubchem.ncbi.nlm.nih.gov | |
Description | Data deposited in or computed by PubChem | |
CAS No. |
28320-73-2 | |
Record name | BOC-GLY-GLY-GLY-OH | |
Source | EPA DSSTox | |
URL | https://comptox.epa.gov/dashboard/DTXSID20427058 | |
Description | DSSTox provides a high quality public chemistry resource for supporting improved predictive toxicology. | |
Synthesis routes and methods
Procedure details
Retrosynthesis Analysis
AI-Powered Synthesis Planning: Our tool employs the Template_relevance Pistachio, Template_relevance Bkms_metabolic, Template_relevance Pistachio_ringbreaker, Template_relevance Reaxys, Template_relevance Reaxys_biocatalysis model, leveraging a vast database of chemical reactions to predict feasible synthetic routes.
One-Step Synthesis Focus: Specifically designed for one-step synthesis, it provides concise and direct routes for your target compounds, streamlining the synthesis process.
Accurate Predictions: Utilizing the extensive PISTACHIO, BKMS_METABOLIC, PISTACHIO_RINGBREAKER, REAXYS, REAXYS_BIOCATALYSIS database, our tool offers high-accuracy predictions, reflecting the latest in chemical research and data.
Strategy Settings
Precursor scoring | Relevance Heuristic |
---|---|
Min. plausibility | 0.01 |
Model | Template_relevance |
Template Set | Pistachio/Bkms_metabolic/Pistachio_ringbreaker/Reaxys/Reaxys_biocatalysis |
Top-N result to add to graph | 6 |
Feasible Synthetic Routes
Disclaimer and Information on In-Vitro Research Products
Please be aware that all articles and product information presented on BenchChem are intended solely for informational purposes. The products available for purchase on BenchChem are specifically designed for in-vitro studies, which are conducted outside of living organisms. In-vitro studies, derived from the Latin term "in glass," involve experiments performed in controlled laboratory settings using cells or tissues. It is important to note that these products are not categorized as medicines or drugs, and they have not received approval from the FDA for the prevention, treatment, or cure of any medical condition, ailment, or disease. We must emphasize that any form of bodily introduction of these products into humans or animals is strictly prohibited by law. It is essential to adhere to these guidelines to ensure compliance with legal and ethical standards in research and experimentation.