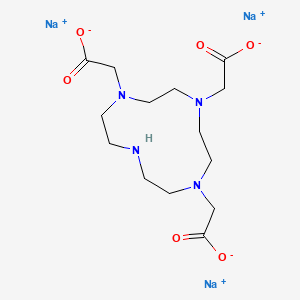
Sodium 2,2',2''-(1,4,7,10-tetraazacyclododecane-1,4,7-triyl)triacetate
Overview
Description
Sodium 2,2',2''-(1,4,7,10-tetraazacyclododecane-1,4,7-triyl)triacetate is a useful research compound. Its molecular formula is C14H23N4Na3O6 and its molecular weight is 412.32 g/mol. The purity is usually 95%.
The exact mass of the compound this compound is unknown and the complexity rating of the compound is unknown. The United Nations designated GHS hazard class pictogram is Irritant, and the GHS signal word is WarningThe storage condition is unknown. Please store according to label instructions upon receipt of goods.
BenchChem offers high-quality this compound suitable for many research applications. Different packaging options are available to accommodate customers' requirements. Please inquire for more information about this compound including the price, delivery time, and more detailed information at info@benchchem.com.
Mechanism of Action
Target of Action
DO3A is a metal chelating agent . Its primary targets are metal ions, particularly gadolinium ions (Gd^3+) . These ions play a crucial role in magnetic resonance imaging (MRI), where they enhance the contrast of the images .
Mode of Action
DO3A forms a tight chelate complex with metal ions such as Gd^3+ . This interaction results in the formation of Gd-DO3A complexes . The chelation process involves the donation of electron pairs from the DO3A to the metal ion, resulting in a stable complex .
Biochemical Pathways
Instead, it influences the magnetic properties of water protons in the vicinity of the complex, which is detected in MRI scans .
Result of Action
The formation of the Gd-DO3A complex enhances the contrast in MRI scans . This allows for clearer visualization of internal body structures, aiding in the diagnosis and monitoring of various medical conditions .
Biochemical Analysis
Biochemical Properties
Sodium 2,2’,2’'-(1,4,7,10-tetraazacyclododecane-1,4,7-triyl)triacetate plays a crucial role in biochemical reactions due to its ability to form stable complexes with metal ions. It interacts with enzymes, proteins, and other biomolecules primarily through chelation. The compound’s strong binding affinity for metal ions allows it to modulate the activity of metalloenzymes and influence various biochemical pathways . For instance, it can form complexes with gadolinium ions, which are used as contrast agents in MRI, enhancing the imaging of biological tissues .
Cellular Effects
The effects of Sodium 2,2’,2’'-(1,4,7,10-tetraazacyclododecane-1,4,7-triyl)triacetate on cells and cellular processes are significant. This compound can influence cell function by altering cell signaling pathways, gene expression, and cellular metabolism. Its ability to chelate metal ions can affect the availability of essential cofactors for enzymatic reactions, thereby modulating metabolic activities . Additionally, the compound’s interaction with cellular proteins can lead to changes in gene expression, impacting various cellular functions .
Molecular Mechanism
At the molecular level, Sodium 2,2’,2’'-(1,4,7,10-tetraazacyclododecane-1,4,7-triyl)triacetate exerts its effects through binding interactions with biomolecules. The compound’s chelation of metal ions can inhibit or activate metalloenzymes, depending on the specific enzyme and metal ion involved . This interaction can lead to changes in the enzyme’s conformation and activity, ultimately affecting biochemical pathways and cellular functions. Additionally, the compound can influence gene expression by modulating the availability of metal ions required for transcription factors and other regulatory proteins .
Temporal Effects in Laboratory Settings
In laboratory settings, the effects of Sodium 2,2’,2’'-(1,4,7,10-tetraazacyclododecane-1,4,7-triyl)triacetate can change over time. The compound’s stability and degradation are critical factors that influence its long-term effects on cellular function. Studies have shown that the compound remains stable under inert atmosphere and at low temperatures (2-8°C), ensuring its efficacy in long-term experiments . Prolonged exposure to environmental factors such as light and air can lead to degradation, affecting its biochemical properties and interactions .
Dosage Effects in Animal Models
The effects of Sodium 2,2’,2’'-(1,4,7,10-tetraazacyclododecane-1,4,7-triyl)triacetate vary with different dosages in animal models. At low doses, the compound can effectively modulate biochemical pathways without causing adverse effects . At high doses, it may exhibit toxic effects, including disruption of cellular functions and metabolic activities . Threshold effects have been observed, where the compound’s efficacy plateaus beyond a certain dosage, indicating the importance of optimizing dosage for therapeutic applications .
Metabolic Pathways
Sodium 2,2’,2’'-(1,4,7,10-tetraazacyclododecane-1,4,7-triyl)triacetate is involved in various metabolic pathways, primarily through its interaction with metalloenzymes and cofactors. The compound’s chelation of metal ions can influence metabolic flux and metabolite levels by modulating enzyme activity . For example, its interaction with gadolinium ions can affect the metabolism of contrast agents used in MRI, enhancing their imaging properties .
Transport and Distribution
Within cells and tissues, Sodium 2,2’,2’'-(1,4,7,10-tetraazacyclododecane-1,4,7-triyl)triacetate is transported and distributed through interactions with transporters and binding proteins. These interactions facilitate the compound’s localization and accumulation in specific cellular compartments . The compound’s ability to form stable complexes with metal ions also influences its distribution, as these complexes can be transported across cell membranes and into target tissues .
Subcellular Localization
The subcellular localization of Sodium 2,2’,2’'-(1,4,7,10-tetraazacyclododecane-1,4,7-triyl)triacetate is determined by its interactions with targeting signals and post-translational modifications. These interactions direct the compound to specific compartments or organelles, where it can exert its biochemical effects . For instance, the compound’s chelation of metal ions can target it to mitochondria, influencing mitochondrial function and metabolic activities .
Properties
IUPAC Name |
trisodium;2-[4,7-bis(carboxylatomethyl)-1,4,7,10-tetrazacyclododec-1-yl]acetate | |
---|---|---|
Source | PubChem | |
URL | https://pubchem.ncbi.nlm.nih.gov | |
Description | Data deposited in or computed by PubChem | |
InChI |
InChI=1S/C14H26N4O6.3Na/c19-12(20)9-16-3-1-15-2-4-17(10-13(21)22)6-8-18(7-5-16)11-14(23)24;;;/h15H,1-11H2,(H,19,20)(H,21,22)(H,23,24);;;/q;3*+1/p-3 | |
Source | PubChem | |
URL | https://pubchem.ncbi.nlm.nih.gov | |
Description | Data deposited in or computed by PubChem | |
InChI Key |
OKHVSTSMWHWVMK-UHFFFAOYSA-K | |
Source | PubChem | |
URL | https://pubchem.ncbi.nlm.nih.gov | |
Description | Data deposited in or computed by PubChem | |
Canonical SMILES |
C1CN(CCN(CCN(CCN1)CC(=O)[O-])CC(=O)[O-])CC(=O)[O-].[Na+].[Na+].[Na+] | |
Source | PubChem | |
URL | https://pubchem.ncbi.nlm.nih.gov | |
Description | Data deposited in or computed by PubChem | |
Molecular Formula |
C14H23N4Na3O6 | |
Source | PubChem | |
URL | https://pubchem.ncbi.nlm.nih.gov | |
Description | Data deposited in or computed by PubChem | |
Molecular Weight |
412.32 g/mol | |
Source | PubChem | |
URL | https://pubchem.ncbi.nlm.nih.gov | |
Description | Data deposited in or computed by PubChem | |
CAS No. |
217973-03-0 | |
Record name | 1,4,7,10-Tetraazacyclododecane-1,4,7-triacetic acid trisodium salt | |
Source | European Chemicals Agency (ECHA) | |
URL | https://echa.europa.eu/information-on-chemicals | |
Description | The European Chemicals Agency (ECHA) is an agency of the European Union which is the driving force among regulatory authorities in implementing the EU's groundbreaking chemicals legislation for the benefit of human health and the environment as well as for innovation and competitiveness. | |
Explanation | Use of the information, documents and data from the ECHA website is subject to the terms and conditions of this Legal Notice, and subject to other binding limitations provided for under applicable law, the information, documents and data made available on the ECHA website may be reproduced, distributed and/or used, totally or in part, for non-commercial purposes provided that ECHA is acknowledged as the source: "Source: European Chemicals Agency, http://echa.europa.eu/". Such acknowledgement must be included in each copy of the material. ECHA permits and encourages organisations and individuals to create links to the ECHA website under the following cumulative conditions: Links can only be made to webpages that provide a link to the Legal Notice page. | |
Record name | Trisodium 1,4,7,10-tetraazacyclododecane-1,4,7-triacetate | |
Source | FDA Global Substance Registration System (GSRS) | |
URL | https://gsrs.ncats.nih.gov/ginas/app/beta/substances/7B6Z3HP8UY | |
Description | The FDA Global Substance Registration System (GSRS) enables the efficient and accurate exchange of information on what substances are in regulated products. Instead of relying on names, which vary across regulatory domains, countries, and regions, the GSRS knowledge base makes it possible for substances to be defined by standardized, scientific descriptions. | |
Explanation | Unless otherwise noted, the contents of the FDA website (www.fda.gov), both text and graphics, are not copyrighted. They are in the public domain and may be republished, reprinted and otherwise used freely by anyone without the need to obtain permission from FDA. Credit to the U.S. Food and Drug Administration as the source is appreciated but not required. | |
Synthesis routes and methods
Procedure details
Disclaimer and Information on In-Vitro Research Products
Please be aware that all articles and product information presented on BenchChem are intended solely for informational purposes. The products available for purchase on BenchChem are specifically designed for in-vitro studies, which are conducted outside of living organisms. In-vitro studies, derived from the Latin term "in glass," involve experiments performed in controlled laboratory settings using cells or tissues. It is important to note that these products are not categorized as medicines or drugs, and they have not received approval from the FDA for the prevention, treatment, or cure of any medical condition, ailment, or disease. We must emphasize that any form of bodily introduction of these products into humans or animals is strictly prohibited by law. It is essential to adhere to these guidelines to ensure compliance with legal and ethical standards in research and experimentation.