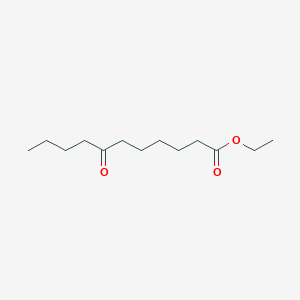
Ethyl 7-oxoundecanoate
- Click on QUICK INQUIRY to receive a quote from our team of experts.
- With the quality product at a COMPETITIVE price, you can focus more on your research.
Overview
Description
Ethyl 7-oxoundecanoate is an organic compound with the molecular formula C₁₃H₂₄O₃ It is a derivative of undecanoic acid, where the seventh carbon atom is oxidized to a ketone group, and the terminal carboxylic acid is esterified with ethanol
Preparation Methods
Synthetic Routes and Reaction Conditions: Ethyl 7-oxoundecanoate can be synthesized through several methods. One common approach involves the oxidation of undecanoic acid to form 7-oxoundecanoic acid, followed by esterification with ethanol. The oxidation step typically employs oxidizing agents such as potassium permanganate or chromium trioxide under controlled conditions. The esterification process can be catalyzed by acid catalysts like sulfuric acid or p-toluenesulfonic acid, with the reaction being carried out under reflux conditions to ensure complete conversion.
Industrial Production Methods: In an industrial setting, the production of this compound may involve continuous flow reactors to optimize reaction efficiency and yield. The use of environmentally friendly oxidizing agents and catalysts is preferred to minimize waste and reduce environmental impact. Advanced purification techniques, such as distillation and recrystallization, are employed to obtain high-purity this compound suitable for various applications.
Chemical Reactions Analysis
Types of Reactions: Ethyl 7-oxoundecanoate undergoes several types of chemical reactions, including:
Oxidation: The ketone group can be further oxidized to form carboxylic acids using strong oxidizing agents.
Reduction: The ketone group can be reduced to a secondary alcohol using reducing agents like sodium borohydride or lithium aluminum hydride.
Substitution: The ester group can undergo nucleophilic substitution reactions, where the ethoxy group is replaced by other nucleophiles.
Common Reagents and Conditions:
Oxidation: Potassium permanganate, chromium trioxide, and hydrogen peroxide under acidic or basic conditions.
Reduction: Sodium borohydride, lithium aluminum hydride, and catalytic hydrogenation.
Substitution: Nucleophiles such as amines, thiols, and alcohols in the presence of acid or base catalysts.
Major Products Formed:
Oxidation: 7-oxoundecanoic acid or 7-hydroxyundecanoic acid.
Reduction: 7-hydroxyundecanoate.
Substitution: Various substituted esters depending on the nucleophile used.
Scientific Research Applications
Ethyl 7-oxoundecanoate has several applications in scientific research, including:
Chemistry: Used as an intermediate in the synthesis of complex organic molecules and as a reagent in organic reactions.
Biology: Studied for its potential role in biological pathways and as a model compound for understanding ester and ketone reactivity.
Medicine: Investigated for its potential therapeutic properties and as a precursor for the synthesis of pharmaceutical compounds.
Industry: Utilized in the production of fragrances, flavors, and as a plasticizer in polymer chemistry.
Mechanism of Action
The mechanism of action of ethyl 7-oxoundecanoate involves its interaction with various molecular targets and pathways. The ketone group can participate in nucleophilic addition reactions, while the ester group can undergo hydrolysis to release the corresponding acid and alcohol. These reactions can modulate biological pathways, making this compound a valuable tool in biochemical studies.
Comparison with Similar Compounds
Ethyl 7-oxoundecanoate can be compared with other similar compounds such as:
Ethyl undecanoate: Lacks the ketone group, making it less reactive in certain chemical reactions.
7-oxoundecanoic acid: The free acid form, which is more reactive in esterification and amidation reactions.
Ethyl 7-hydroxyundecanoate: Contains a hydroxyl group instead of a ketone, leading to different reactivity and applications.
This compound stands out due to its unique combination of a ketone and ester functional group, providing a versatile platform for various chemical transformations and applications.
Properties
IUPAC Name |
ethyl 7-oxoundecanoate |
Source
|
---|---|---|
Source | PubChem | |
URL | https://pubchem.ncbi.nlm.nih.gov | |
Description | Data deposited in or computed by PubChem | |
InChI |
InChI=1S/C13H24O3/c1-3-5-9-12(14)10-7-6-8-11-13(15)16-4-2/h3-11H2,1-2H3 |
Source
|
Source | PubChem | |
URL | https://pubchem.ncbi.nlm.nih.gov | |
Description | Data deposited in or computed by PubChem | |
InChI Key |
VCUXSPMIROUACC-UHFFFAOYSA-N |
Source
|
Source | PubChem | |
URL | https://pubchem.ncbi.nlm.nih.gov | |
Description | Data deposited in or computed by PubChem | |
Canonical SMILES |
CCCCC(=O)CCCCCC(=O)OCC |
Source
|
Source | PubChem | |
URL | https://pubchem.ncbi.nlm.nih.gov | |
Description | Data deposited in or computed by PubChem | |
Molecular Formula |
C13H24O3 |
Source
|
Source | PubChem | |
URL | https://pubchem.ncbi.nlm.nih.gov | |
Description | Data deposited in or computed by PubChem | |
DSSTOX Substance ID |
DTXSID90444055 |
Source
|
Record name | Ethyl 7-oxoundecanoate | |
Source | EPA DSSTox | |
URL | https://comptox.epa.gov/dashboard/DTXSID90444055 | |
Description | DSSTox provides a high quality public chemistry resource for supporting improved predictive toxicology. | |
Molecular Weight |
228.33 g/mol |
Source
|
Source | PubChem | |
URL | https://pubchem.ncbi.nlm.nih.gov | |
Description | Data deposited in or computed by PubChem | |
CAS No. |
227953-80-2 |
Source
|
Record name | Ethyl 7-oxoundecanoate | |
Source | EPA DSSTox | |
URL | https://comptox.epa.gov/dashboard/DTXSID90444055 | |
Description | DSSTox provides a high quality public chemistry resource for supporting improved predictive toxicology. | |
Disclaimer and Information on In-Vitro Research Products
Please be aware that all articles and product information presented on BenchChem are intended solely for informational purposes. The products available for purchase on BenchChem are specifically designed for in-vitro studies, which are conducted outside of living organisms. In-vitro studies, derived from the Latin term "in glass," involve experiments performed in controlled laboratory settings using cells or tissues. It is important to note that these products are not categorized as medicines or drugs, and they have not received approval from the FDA for the prevention, treatment, or cure of any medical condition, ailment, or disease. We must emphasize that any form of bodily introduction of these products into humans or animals is strictly prohibited by law. It is essential to adhere to these guidelines to ensure compliance with legal and ethical standards in research and experimentation.