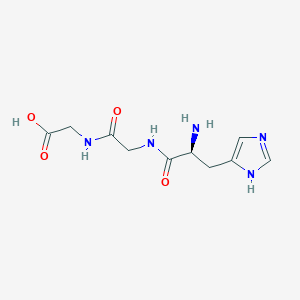
Glycine, L-histidylglycyl-
Description
Glycine, L-histidylglycyl- is a dipeptide composed of glycine and L-histidine. It is a small molecule with significant biological and chemical importance. This compound is known for its role in various biochemical processes and its potential therapeutic applications in medicine.
Synthetic Routes and Reaction Conditions:
Ammonolysis of Chloroacetic Acid: This method involves the reaction of chloroacetic acid with ammonia in the presence of a catalyst such as urotropine.
Strecker Synthesis: This method involves the reaction of formaldehyde, ammonium chloride, and sodium cyanide to form amino acetonitrile, which is then hydrolyzed to produce glycine.
Industrial Production Methods:
Biotechnological Synthesis: This method utilizes microbial fermentation to produce glycine. Specific strains of bacteria or yeast are engineered to overproduce glycine, which is
Properties
IUPAC Name |
2-[[2-[[(2S)-2-amino-3-(1H-imidazol-5-yl)propanoyl]amino]acetyl]amino]acetic acid | |
---|---|---|
Source | PubChem | |
URL | https://pubchem.ncbi.nlm.nih.gov | |
Description | Data deposited in or computed by PubChem | |
InChI |
InChI=1S/C10H15N5O4/c11-7(1-6-2-12-5-15-6)10(19)14-3-8(16)13-4-9(17)18/h2,5,7H,1,3-4,11H2,(H,12,15)(H,13,16)(H,14,19)(H,17,18)/t7-/m0/s1 | |
Source | PubChem | |
URL | https://pubchem.ncbi.nlm.nih.gov | |
Description | Data deposited in or computed by PubChem | |
InChI Key |
FDQYIRHBVVUTJF-ZETCQYMHSA-N | |
Source | PubChem | |
URL | https://pubchem.ncbi.nlm.nih.gov | |
Description | Data deposited in or computed by PubChem | |
Canonical SMILES |
C1=C(NC=N1)CC(C(=O)NCC(=O)NCC(=O)O)N | |
Source | PubChem | |
URL | https://pubchem.ncbi.nlm.nih.gov | |
Description | Data deposited in or computed by PubChem | |
Isomeric SMILES |
C1=C(NC=N1)C[C@@H](C(=O)NCC(=O)NCC(=O)O)N | |
Source | PubChem | |
URL | https://pubchem.ncbi.nlm.nih.gov | |
Description | Data deposited in or computed by PubChem | |
Molecular Formula |
C10H15N5O4 | |
Source | PubChem | |
URL | https://pubchem.ncbi.nlm.nih.gov | |
Description | Data deposited in or computed by PubChem | |
DSSTOX Substance ID |
DTXSID20427250 | |
Record name | Glycine, L-histidylglycyl- | |
Source | EPA DSSTox | |
URL | https://comptox.epa.gov/dashboard/DTXSID20427250 | |
Description | DSSTox provides a high quality public chemistry resource for supporting improved predictive toxicology. | |
Molecular Weight |
269.26 g/mol | |
Source | PubChem | |
URL | https://pubchem.ncbi.nlm.nih.gov | |
Description | Data deposited in or computed by PubChem | |
CAS No. |
32999-80-7 | |
Record name | Glycine, L-histidylglycyl- | |
Source | EPA DSSTox | |
URL | https://comptox.epa.gov/dashboard/DTXSID20427250 | |
Description | DSSTox provides a high quality public chemistry resource for supporting improved predictive toxicology. | |
Retrosynthesis Analysis
AI-Powered Synthesis Planning: Our tool employs the Template_relevance Pistachio, Template_relevance Bkms_metabolic, Template_relevance Pistachio_ringbreaker, Template_relevance Reaxys, Template_relevance Reaxys_biocatalysis model, leveraging a vast database of chemical reactions to predict feasible synthetic routes.
One-Step Synthesis Focus: Specifically designed for one-step synthesis, it provides concise and direct routes for your target compounds, streamlining the synthesis process.
Accurate Predictions: Utilizing the extensive PISTACHIO, BKMS_METABOLIC, PISTACHIO_RINGBREAKER, REAXYS, REAXYS_BIOCATALYSIS database, our tool offers high-accuracy predictions, reflecting the latest in chemical research and data.
Strategy Settings
Precursor scoring | Relevance Heuristic |
---|---|
Min. plausibility | 0.01 |
Model | Template_relevance |
Template Set | Pistachio/Bkms_metabolic/Pistachio_ringbreaker/Reaxys/Reaxys_biocatalysis |
Top-N result to add to graph | 6 |
Feasible Synthetic Routes
Q1: What is the molecular formula and weight of His-Gly-Gly?
A1: The molecular formula of His-Gly-Gly is C8H12N6O4. Its molecular weight is 240.22 g/mol.
Q2: Is there spectroscopic data available for His-Gly-Gly?
A2: Yes, various spectroscopic techniques have been employed to study His-Gly-Gly. [1H-NMR studies have provided insights into the interaction of His-Gly-Gly with Zinc (II) ions] []. [Raman Optical Activity (ROA) spectroscopy has been used to probe the binding of lanthanide complexes to His-Gly-Gly] [].
Q3: How does His-Gly-Gly interact with metal ions?
A3: His-Gly-Gly readily forms complexes with various metal ions, including copper (II) and zinc (II) [, , , ]. [The histidine residue in His-Gly-Gly plays a crucial role in metal ion coordination due to the presence of the imidazole ring] [].
Q4: What is the significance of copper binding to His-Gly-Gly in the context of prion proteins?
A5: [His-Gly-Gly is structurally similar to the octapeptide repeat found in the N-terminal region of the prion protein] [, , ]. This region is known to bind copper ions and is implicated in the protein's function and potential role in prion diseases [, ]. [Studies suggest that the tryptophan residues within the octarepeat region may play a role in the copper-reducing activity of prion proteins] [].
Q5: How do lanthanide complexes interact with His-Gly-Gly?
A6: [Lanthanide complexes bind relatively weakly to the histidine residue in His-Gly-Gly, with other parts of the peptide also participating in the interaction] []. [Longer peptides, low pH, and a histidine residue closer to the N-terminus tend to favor binding] [].
Q6: What are the potential applications of His-Gly-Gly?
A6: Due to its metal-binding properties, His-Gly-Gly has potential applications in various fields, including:
- Chemical Sensors: His-Gly-Gly immobilized on solid surfaces can act as a selective sensor for copper ions [].
- Biomimetic Catalysis: [Immobilized metal-His-Gly-Gly complexes on mesoporous silica can mimic the active center of methane monooxygenase and exhibit catalytic activity in cyclohexane oxidation] [, ].
- Drug Delivery: [His-Gly-Gly is being explored as a component in sustained-release microsphere formulations for exendin, a drug used in the treatment of type 2 diabetes] [].
Q7: Have computational methods been used to study His-Gly-Gly?
A8: Yes, [computational chemistry techniques, such as density functional theory (DFT) and Car-Parrinello molecular dynamics, have been employed to model the interaction of copper with His-Gly-Gly and related peptides] []. [These simulations provide insights into the structural features and energetics of copper binding to the peptide backbone] [].
Disclaimer and Information on In-Vitro Research Products
Please be aware that all articles and product information presented on BenchChem are intended solely for informational purposes. The products available for purchase on BenchChem are specifically designed for in-vitro studies, which are conducted outside of living organisms. In-vitro studies, derived from the Latin term "in glass," involve experiments performed in controlled laboratory settings using cells or tissues. It is important to note that these products are not categorized as medicines or drugs, and they have not received approval from the FDA for the prevention, treatment, or cure of any medical condition, ailment, or disease. We must emphasize that any form of bodily introduction of these products into humans or animals is strictly prohibited by law. It is essential to adhere to these guidelines to ensure compliance with legal and ethical standards in research and experimentation.