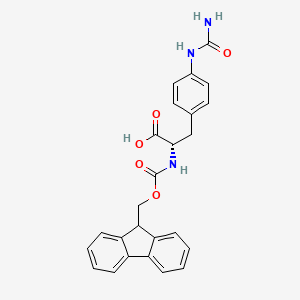
Fmoc-Aph(Cbm)-OH
Overview
Description
Fmoc-Aph(Cbm)-OH, also known as N-(fluorenylmethoxycarbonyl)-4-ureido-L-phenylalanine, is an organic compound with the molecular formula C25H23N3O5 . It is a type of amino acid protecting group, often used in peptide synthesis and solid-phase synthesis .
Synthesis Analysis
The synthesis of this compound is complex and usually involves multiple steps and synthesis . A common synthesis method involves the reaction of 2-phenylmethyl oxirane (Fmoc-Cbm-OH) with D-phenylalanine (D-Aph-OH) to produce fluorenylmethoxycarbonyl-D-phenylalanine (Fmoc-D-Aph-OH). This is then transformed into its amide form (Fmoc-D-Aph(Cbm)-OH) through an aminocarbonylation reaction .Molecular Structure Analysis
The molecular structure of this compound includes a total of 59 bonds. There are 36 non-H bonds, 21 multiple bonds, 8 rotatable bonds, 3 double bonds, 18 aromatic bonds, 1 five-membered ring, 3 six-membered rings, 2 nine-membered rings, 1 carboxylic acid (aliphatic), 1 (thio-) carbamate (aliphatic), and 1 urea (-thio) derivative .Chemical Reactions Analysis
As an amino acid protecting group, this compound plays a crucial role in peptide synthesis and solid-phase synthesis. It prevents the amino acid from undergoing nonspecific reactions during the synthesis process, thereby protecting the target amino acid .Physical and Chemical Properties Analysis
This compound has a molecular weight of 445.5 g/mol . It has a computed XLogP3-AA value of 3.2, indicating its lipophilicity . It has 4 hydrogen bond donors and 5 hydrogen bond acceptors . The compound has 8 rotatable bonds, contributing to its flexibility . Its exact mass and monoisotopic mass are both 445.16377084 g/mol . The topological polar surface area is 131 Ų .Scientific Research Applications
Solid-Phase Synthesis of Peptides
Fmoc-Aph(Cbm)-OH, with its protective Fmoc group, is instrumental in the solid-phase synthesis of peptides. Šebesta and Seebach (2003) delineated the preparation of N-Fmoc-protected β2-homoamino acids for synthesizing β-peptides via solid-phase methods. Their research demonstrated a method suitable for large-scale preparation of Fmoc-β2hXaa-OH, integral for the solid-phase synthesis of β-peptides. These Fmoc-amino acids and their precursors were comprehensively characterized, laying a foundation for the use of Fmoc-protected amino acids in peptide synthesis (Šebesta & Seebach, 2003).
Development of Protected Amino Acid Oligomers
This compound is also essential in the development of protected amino acid oligomers. Ishibashi and Kitamura (2009) advanced the solid-phase synthesis of a protected form of a new type of unnatural peptide alpha-amino phosphonic acid oligomer (APO) using Fmoc-(GlyP(OBn))(6)-OH/DIEA. This work underscores the versatility of Fmoc-protected amino acids in creating novel oligomers, expanding the horizons of synthetic peptide chemistry (Ishibashi & Kitamura, 2009).
Advancements in Nanotechnology for Biomedical Applications
This compound plays a pivotal role in the burgeoning field of peptide- and amino-acid-based nanotechnology, especially for biomedical applications. Schnaider et al. (2019) discussed the utilization of fluorenylmethyloxycarbonyl (Fmoc)-decorated self-assembling building blocks for antibacterial and anti-inflammatory purposes. The study highlighted the antibacterial capabilities of the nanoassemblies formed by Fmoc-pentafluoro-l-phenylalanine-OH and their integration within resin-based composites, underscoring the potential of Fmoc-protected amino acids in developing advanced biomedical materials (Schnaider et al., 2019).
Mechanism of Action
Target of Action
Fmoc-Aph(Cbm)-OH, a modified amino acid, primarily targets the formation of various structures through self-assembly . The inherent hydrophobicity and aromaticity of the Fmoc moiety promote the association of building blocks .
Mode of Action
The compound interacts with its targets through self-assembly, a process facilitated by the hydrophobic interactions from the fluorenyl ring and steric optimization from the linker (the methoxycarbonyl group) . This results in the formation of various structures that serve as excellent bio-organic scaffolds for diverse applications .
Biochemical Pathways
The biochemical pathways affected by this compound are primarily related to the self-assembly of the compound. The unique interactions, along with the driving forces induced by the peptide sequences, result in the self-assembly of Fmoc-modified amino acids and short peptides in a kinetically rapid and thermodynamically rigid manner .
Result of Action
The result of this compound’s action is the formation of various structures with unique properties . These structures serve as bio-organic scaffolds for diverse applications, including cell cultivation, bio-templating, optical, drug delivery, catalytic, therapeutic, and antibiotic properties .
Action Environment
The action of this compound can be influenced by environmental factors. For instance, solvent variation can control the morphologies resulting from self-assembly . Furthermore, pH-dependent self-assembly can modulate the optical properties of Fmoc .
Future Directions
Properties
IUPAC Name |
(2S)-3-[4-(carbamoylamino)phenyl]-2-(9H-fluoren-9-ylmethoxycarbonylamino)propanoic acid | |
---|---|---|
Source | PubChem | |
URL | https://pubchem.ncbi.nlm.nih.gov | |
Description | Data deposited in or computed by PubChem | |
InChI |
InChI=1S/C25H23N3O5/c26-24(31)27-16-11-9-15(10-12-16)13-22(23(29)30)28-25(32)33-14-21-19-7-3-1-5-17(19)18-6-2-4-8-20(18)21/h1-12,21-22H,13-14H2,(H,28,32)(H,29,30)(H3,26,27,31)/t22-/m0/s1 | |
Source | PubChem | |
URL | https://pubchem.ncbi.nlm.nih.gov | |
Description | Data deposited in or computed by PubChem | |
InChI Key |
HFPDOFBZCSQAEJ-QFIPXVFZSA-N | |
Source | PubChem | |
URL | https://pubchem.ncbi.nlm.nih.gov | |
Description | Data deposited in or computed by PubChem | |
Canonical SMILES |
C1=CC=C2C(=C1)C(C3=CC=CC=C32)COC(=O)NC(CC4=CC=C(C=C4)NC(=O)N)C(=O)O | |
Source | PubChem | |
URL | https://pubchem.ncbi.nlm.nih.gov | |
Description | Data deposited in or computed by PubChem | |
Isomeric SMILES |
C1=CC=C2C(=C1)C(C3=CC=CC=C32)COC(=O)N[C@@H](CC4=CC=C(C=C4)NC(=O)N)C(=O)O | |
Source | PubChem | |
URL | https://pubchem.ncbi.nlm.nih.gov | |
Description | Data deposited in or computed by PubChem | |
Molecular Formula |
C25H23N3O5 | |
Source | PubChem | |
URL | https://pubchem.ncbi.nlm.nih.gov | |
Description | Data deposited in or computed by PubChem | |
DSSTOX Substance ID |
DTXSID001139856 | |
Record name | 4-[(Aminocarbonyl)amino]-N-[(9H-fluoren-9-ylmethoxy)carbonyl]-L-phenylalanine | |
Source | EPA DSSTox | |
URL | https://comptox.epa.gov/dashboard/DTXSID001139856 | |
Description | DSSTox provides a high quality public chemistry resource for supporting improved predictive toxicology. | |
Molecular Weight |
445.5 g/mol | |
Source | PubChem | |
URL | https://pubchem.ncbi.nlm.nih.gov | |
Description | Data deposited in or computed by PubChem | |
CAS No. |
324017-23-4 | |
Record name | 4-[(Aminocarbonyl)amino]-N-[(9H-fluoren-9-ylmethoxy)carbonyl]-L-phenylalanine | |
Source | CAS Common Chemistry | |
URL | https://commonchemistry.cas.org/detail?cas_rn=324017-23-4 | |
Description | CAS Common Chemistry is an open community resource for accessing chemical information. Nearly 500,000 chemical substances from CAS REGISTRY cover areas of community interest, including common and frequently regulated chemicals, and those relevant to high school and undergraduate chemistry classes. This chemical information, curated by our expert scientists, is provided in alignment with our mission as a division of the American Chemical Society. | |
Explanation | The data from CAS Common Chemistry is provided under a CC-BY-NC 4.0 license, unless otherwise stated. | |
Record name | 4-[(Aminocarbonyl)amino]-N-[(9H-fluoren-9-ylmethoxy)carbonyl]-L-phenylalanine | |
Source | EPA DSSTox | |
URL | https://comptox.epa.gov/dashboard/DTXSID001139856 | |
Description | DSSTox provides a high quality public chemistry resource for supporting improved predictive toxicology. | |
Disclaimer and Information on In-Vitro Research Products
Please be aware that all articles and product information presented on BenchChem are intended solely for informational purposes. The products available for purchase on BenchChem are specifically designed for in-vitro studies, which are conducted outside of living organisms. In-vitro studies, derived from the Latin term "in glass," involve experiments performed in controlled laboratory settings using cells or tissues. It is important to note that these products are not categorized as medicines or drugs, and they have not received approval from the FDA for the prevention, treatment, or cure of any medical condition, ailment, or disease. We must emphasize that any form of bodily introduction of these products into humans or animals is strictly prohibited by law. It is essential to adhere to these guidelines to ensure compliance with legal and ethical standards in research and experimentation.