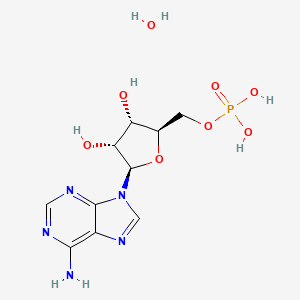
Adenosine 5'-monophosphate monohydrate
Overview
Description
Adenosine 5’-monophosphate monohydrate, also known as 5’-adenylic acid, is a nucleotide that plays a crucial role in various biological processes. It is composed of a phosphate group, the sugar ribose, and the nucleobase adenine. This compound is found in RNA and is involved in energy transfer and signal transduction within cells .
Scientific Research Applications
Adenosine 5’-monophosphate monohydrate has numerous applications in scientific research:
Chemistry: Used as a reagent in the synthesis of other nucleotides and nucleotide analogs.
Biology: Plays a role in signal transduction and energy transfer within cells.
Medicine: Investigated for its potential therapeutic effects in conditions such as ischemia and inflammation.
Industry: Used in the production of flavor enhancers and as a nutritional supplement.
Mechanism of Action
Adenosine 5’-monophosphate monohydrate exerts its effects primarily through the activation of AMP-activated protein kinase (AMPK). This enzyme plays a key role in regulating cellular energy homeostasis. The compound also affects various immune functions, including the enhancement of T-cell maturation and natural killer cell activity .
Similar Compounds:
- Adenosine diphosphate (ADP)
- Adenosine triphosphate (ATP)
- Cytidine monophosphate (CMP)
- Guanosine monophosphate (GMP)
Comparison: Adenosine 5’-monophosphate monohydrate is unique in its role as a precursor to both ADP and ATP, which are critical for energy transfer in cells. Unlike CMP and GMP, which are involved in different metabolic pathways, adenosine 5’-monophosphate monohydrate is specifically linked to energy metabolism and signal transduction .
Safety and Hazards
When handling Adenosine 5’-monophosphate monohydrate, avoid breathing mist, gas, or vapors. Avoid contact with skin and eyes. Use personal protective equipment and wear chemical impermeable gloves. Ensure adequate ventilation. Remove all sources of ignition. Evacuate personnel to safe areas. Keep people away from and upwind of spill/leak .
Future Directions
Adenosine 5’-monophosphate has been used in various studies, such as its rate of hydrolysis in Escherichia coli lysates , inducing calcium responses in Fura2-loaded osteoblast , and as a nucleotide metabolite in the medium for the isolation, culture, and identification of glioma stem cells . It has also been studied for its effects on the growth performance, carcass characteristics, meat quality, and lipid metabolism in adipose tissues of finishing pigs .
Biochemical Analysis
Biochemical Properties
Adenosine 5’-monophosphate monohydrate is an activator of a class of protein kinases known as AMP-activated protein kinase. This enzyme plays a significant role in cellular energy homeostasis by regulating various metabolic pathways. Adenosine 5’-monophosphate monohydrate interacts with enzymes such as AMP-thymidine kinase, AMP deaminase, and 5’-nucleotidase. These interactions are essential for the synthesis and degradation of nucleotides, as well as for the regulation of cellular energy levels .
Cellular Effects
Adenosine 5’-monophosphate monohydrate influences various cellular processes, including cell signaling pathways, gene expression, and cellular metabolism. It is involved in the activation of AMP-activated protein kinase, which regulates glucose uptake, fatty acid oxidation, and mitochondrial biogenesis. This compound also affects immune functions by enhancing T-cell maturation and natural killer cell activity .
Molecular Mechanism
The molecular mechanism of adenosine 5’-monophosphate monohydrate involves its role as an activator of AMP-activated protein kinase. This enzyme is activated by an increase in the AMP to ATP ratio, which occurs during cellular stress or energy depletion. Once activated, AMP-activated protein kinase phosphorylates various downstream targets, leading to the inhibition of anabolic pathways and the activation of catabolic pathways. This results in increased energy production and conservation .
Temporal Effects in Laboratory Settings
In laboratory settings, the effects of adenosine 5’-monophosphate monohydrate can change over time. The compound is relatively stable, but its activity can be influenced by factors such as temperature and pH. Long-term studies have shown that adenosine 5’-monophosphate monohydrate can enhance the activity of certain enzymes, such as 5-oxoprolinase, over extended periods .
Dosage Effects in Animal Models
The effects of adenosine 5’-monophosphate monohydrate vary with different dosages in animal models. At lower doses, it can enhance immune function and improve glucose metabolism. At higher doses, it may cause adverse effects such as toxicity and metabolic imbalances. Studies have shown that the compound can activate AMP-activated protein kinase in skeletal muscle, leading to improved insulin sensitivity and glucose uptake .
Metabolic Pathways
Adenosine 5’-monophosphate monohydrate is involved in several metabolic pathways, including the purine nucleotide cycle and the synthesis of RNA. It can be converted to inosine monophosphate by the enzyme myoadenylate deaminase, which is then further metabolized to uric acid for excretion. This compound also plays a role in the regulation of energy metabolism by influencing the levels of ATP and ADP .
Transport and Distribution
Within cells, adenosine 5’-monophosphate monohydrate is transported and distributed by various transporters and binding proteins. It can be taken up by cells through nucleoside transporters and is distributed to different cellular compartments where it exerts its effects. The compound’s localization and accumulation can be influenced by factors such as cellular energy status and the presence of specific binding proteins .
Subcellular Localization
Adenosine 5’-monophosphate monohydrate is localized in various subcellular compartments, including the cytoplasm and mitochondria. Its activity and function can be affected by its subcellular localization, as well as by post-translational modifications that direct it to specific organelles. For example, the activation of AMP-activated protein kinase by adenosine 5’-monophosphate monohydrate occurs primarily in the cytoplasm, where it regulates metabolic pathways .
Preparation Methods
Synthetic Routes and Reaction Conditions: Adenosine 5’-monophosphate monohydrate can be synthesized through enzymatic reactions involving adenosine and phosphate donors. One common method involves the use of adenosine kinase to phosphorylate adenosine in the presence of adenosine triphosphate (ATP) as a phosphate donor .
Industrial Production Methods: Industrial production of adenosine 5’-monophosphate monohydrate often involves microbial fermentation. Microorganisms such as Candida utilis are used to produce the compound, which is then extracted and purified through various chromatographic techniques .
Chemical Reactions Analysis
Types of Reactions: Adenosine 5’-monophosphate monohydrate undergoes several types of chemical reactions, including:
Phosphorylation: Conversion to adenosine diphosphate (ADP) and adenosine triphosphate (ATP).
Dephosphorylation: Conversion back to adenosine.
Hydrolysis: Breakdown into adenosine and inorganic phosphate.
Common Reagents and Conditions:
Phosphorylation: Requires adenosine kinase and ATP.
Dephosphorylation: Catalyzed by phosphatases.
Hydrolysis: Typically occurs under acidic or enzymatic conditions.
Major Products:
Phosphorylation: ADP and ATP.
Dephosphorylation: Adenosine.
Hydrolysis: Adenosine and inorganic phosphate.
Properties
IUPAC Name |
[(2R,3S,4R,5R)-5-(6-aminopurin-9-yl)-3,4-dihydroxyoxolan-2-yl]methyl dihydrogen phosphate;hydrate | |
---|---|---|
Source | PubChem | |
URL | https://pubchem.ncbi.nlm.nih.gov | |
Description | Data deposited in or computed by PubChem | |
InChI |
InChI=1S/C10H14N5O7P.H2O/c11-8-5-9(13-2-12-8)15(3-14-5)10-7(17)6(16)4(22-10)1-21-23(18,19)20;/h2-4,6-7,10,16-17H,1H2,(H2,11,12,13)(H2,18,19,20);1H2/t4-,6-,7-,10-;/m1./s1 | |
Source | PubChem | |
URL | https://pubchem.ncbi.nlm.nih.gov | |
Description | Data deposited in or computed by PubChem | |
InChI Key |
ZOEFQKVADUBYKV-MCDZGGTQSA-N | |
Source | PubChem | |
URL | https://pubchem.ncbi.nlm.nih.gov | |
Description | Data deposited in or computed by PubChem | |
Canonical SMILES |
C1=NC(=C2C(=N1)N(C=N2)C3C(C(C(O3)COP(=O)(O)O)O)O)N.O | |
Source | PubChem | |
URL | https://pubchem.ncbi.nlm.nih.gov | |
Description | Data deposited in or computed by PubChem | |
Isomeric SMILES |
C1=NC(=C2C(=N1)N(C=N2)[C@H]3[C@@H]([C@@H]([C@H](O3)COP(=O)(O)O)O)O)N.O | |
Source | PubChem | |
URL | https://pubchem.ncbi.nlm.nih.gov | |
Description | Data deposited in or computed by PubChem | |
Molecular Formula |
C10H16N5O8P | |
Source | PubChem | |
URL | https://pubchem.ncbi.nlm.nih.gov | |
Description | Data deposited in or computed by PubChem | |
DSSTOX Substance ID |
DTXSID00939806 | |
Record name | 9-(5-O-Phosphonopentofuranosyl)-9H-purin-6-amine--water (1/1) | |
Source | EPA DSSTox | |
URL | https://comptox.epa.gov/dashboard/DTXSID00939806 | |
Description | DSSTox provides a high quality public chemistry resource for supporting improved predictive toxicology. | |
Molecular Weight |
365.24 g/mol | |
Source | PubChem | |
URL | https://pubchem.ncbi.nlm.nih.gov | |
Description | Data deposited in or computed by PubChem | |
CAS No. |
18422-05-4 | |
Record name | 9-(5-O-Phosphonopentofuranosyl)-9H-purin-6-amine--water (1/1) | |
Source | EPA DSSTox | |
URL | https://comptox.epa.gov/dashboard/DTXSID00939806 | |
Description | DSSTox provides a high quality public chemistry resource for supporting improved predictive toxicology. | |
Retrosynthesis Analysis
AI-Powered Synthesis Planning: Our tool employs the Template_relevance Pistachio, Template_relevance Bkms_metabolic, Template_relevance Pistachio_ringbreaker, Template_relevance Reaxys, Template_relevance Reaxys_biocatalysis model, leveraging a vast database of chemical reactions to predict feasible synthetic routes.
One-Step Synthesis Focus: Specifically designed for one-step synthesis, it provides concise and direct routes for your target compounds, streamlining the synthesis process.
Accurate Predictions: Utilizing the extensive PISTACHIO, BKMS_METABOLIC, PISTACHIO_RINGBREAKER, REAXYS, REAXYS_BIOCATALYSIS database, our tool offers high-accuracy predictions, reflecting the latest in chemical research and data.
Strategy Settings
Precursor scoring | Relevance Heuristic |
---|---|
Min. plausibility | 0.01 |
Model | Template_relevance |
Template Set | Pistachio/Bkms_metabolic/Pistachio_ringbreaker/Reaxys/Reaxys_biocatalysis |
Top-N result to add to graph | 6 |
Feasible Synthetic Routes
Q1: How does Adenosine 5'-monophosphate monohydrate interact with its target in the Zika virus, and what are the downstream effects?
A1: Research suggests that this compound targets the Zika virus NS5 methyltransferase, similar to the native inhibitor S-adenosylhomocysteine (SAH) []. This interaction likely occurs through stable binding at the enzyme's active site, potentially mimicking SAH and disrupting the methylation processes crucial for viral replication []. Further research is needed to confirm the exact mechanism of action and downstream effects on viral replication.
Q2: What is the structural characterization of this compound, including its molecular formula and weight?
A2: this compound, also known as Adenosine monophosphate (AMP), has the molecular formula C10H14N5O7P·H2O. Its molecular weight is 365.24 g/mol. Information on specific spectroscopic data was not provided in the analyzed research papers.
Q3: Has this compound been investigated using computational chemistry methods?
A3: Yes, computational chemistry played a key role in identifying this compound as a potential Zika virus inhibitor. Researchers employed various techniques like molecular docking, molecular dynamics (MD) simulations, and calculations of binding free energies using methods like MM/GBSA []. These simulations provided insights into the binding stability of this compound with the Zika virus NS5 methyltransferase and its potential as an inhibitor [].
Disclaimer and Information on In-Vitro Research Products
Please be aware that all articles and product information presented on BenchChem are intended solely for informational purposes. The products available for purchase on BenchChem are specifically designed for in-vitro studies, which are conducted outside of living organisms. In-vitro studies, derived from the Latin term "in glass," involve experiments performed in controlled laboratory settings using cells or tissues. It is important to note that these products are not categorized as medicines or drugs, and they have not received approval from the FDA for the prevention, treatment, or cure of any medical condition, ailment, or disease. We must emphasize that any form of bodily introduction of these products into humans or animals is strictly prohibited by law. It is essential to adhere to these guidelines to ensure compliance with legal and ethical standards in research and experimentation.