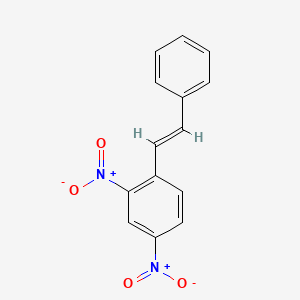
2,4-Dinitrostilbene
Overview
Description
2,4-Dinitrostilbene is an organic compound belonging to the stilbene family, characterized by the presence of two nitro groups attached to the stilbene backbone. Stilbenes are a group of compounds known for their diverse biological activities and applications in various fields, including pharmaceuticals and material science .
Preparation Methods
Synthetic Routes and Reaction Conditions: 2,4-Dinitrostilbene can be synthesized through several methods. One common approach involves the reaction of 4-nitrobenzyl bromide with 4-nitrobenzaldehyde, followed by a reduction step using stannous chloride (SnCl2) to yield this compound . Another method involves the oxidative dehydrogenation of nitro derivatives of bibenzyl using a mixture of 2,2,6,6-tetramethylpiperidine-1-oxyl (TEMPO) and metal salts under atmospheric oxygen .
Industrial Production Methods: In industrial settings, the production of this compound often involves the sulfonation of 4-nitrotoluene to produce 4-nitrotoluene-2-sulfonic acid, followed by oxidation with sodium hypochlorite to yield the desired compound .
Chemical Reactions Analysis
Types of Reactions: 2,4-Dinitrostilbene undergoes various chemical reactions, including:
Oxidation: The compound can be oxidized to form corresponding nitro stilbenes.
Reduction: Reduction of the nitro groups can yield amino derivatives.
Substitution: The nitro groups can be substituted with other functional groups under appropriate conditions
Common Reagents and Conditions:
Oxidation: TEMPO and metal salts under atmospheric oxygen.
Reduction: Stannous chloride (SnCl2).
Substitution: Various nucleophiles under basic or acidic conditions.
Major Products:
Oxidation: Nitro stilbenes.
Reduction: Amino stilbenes.
Substitution: Substituted stilbenes with different functional groups
Scientific Research Applications
Cancer Research
2,4-Dinitrostilbene has been investigated for its antiproliferative effects on cancer cell lines. Studies have shown that stilbene derivatives can inhibit cell growth in various cancer types:
- Cell Lines Tested :
- HCT116 (colon carcinoma)
- MCF-7 (breast adenocarcinoma)
- U87 (glioblastoma)
- HeLa (cervical cancer)
Research indicates that certain stilbene derivatives exhibit significant cytotoxicity against these cancer cell lines, with varying degrees of selectivity. For instance, some compounds showed a half-maximal inhibitory concentration (IC50) below 30 µM, indicating strong antiproliferative activity .
Compound | Cell Line | IC50 (µM) | Selectivity Index |
---|---|---|---|
1a | HCT116 | <30 | 1.4 |
1c | MCF-7 | <30 | 2.0 |
1d | U87 | <30 | 2.4 |
Material Science
In material science, this compound has been explored for its potential as a fluorescent probe and dye. Its ability to absorb light and emit fluorescence makes it useful in various applications such as:
- Fluorescent Markers : Used in biological imaging techniques.
- Polymer Chemistry : Acts as a sensitizer in photopolymerization processes.
The compound's unique photophysical properties enable it to be incorporated into polymers to enhance their optical characteristics.
Analytical Chemistry
This compound is utilized as a reagent in analytical chemistry for the detection and quantification of various analytes:
- Chromatographic Techniques : Employed in High-Performance Liquid Chromatography (HPLC) for separation and analysis.
- Spectroscopic Applications : Used as a standard in fluorescence spectroscopy due to its well-defined spectral properties.
Case Studies
Several studies highlight the effectiveness of this compound in practical applications:
- A study demonstrated that stilbene derivatives could inhibit tubulin polymerization, which is crucial for cancer cell proliferation. This mechanism suggests that these compounds may serve as potential chemotherapeutic agents targeting microtubule dynamics .
- Another investigation focused on the synthesis of new stilbene derivatives that showed enhanced activity against resistant cancer cell lines, indicating the compound's versatility and potential for drug development .
Mechanism of Action
The mechanism of action of 2,4-Dinitrostilbene involves its interaction with molecular targets and pathways. For instance, its anticancer properties are attributed to its ability to interact with tubulin, leading to the disruption of the microtubular cytoskeleton, which is essential for cell division . Additionally, its antimicrobial activity is linked to its ability to interfere with bacterial cell wall synthesis .
Comparison with Similar Compounds
Resveratrol: A naturally occurring stilbene with antioxidant and anticancer properties.
Pterostilbene: Known for its antitumor and antidiabetic activities.
Combretastatin A-4: Exhibits potent anticancer activity by targeting tubulin.
Uniqueness: 2,4-Dinitrostilbene is unique due to its specific nitro substitutions, which confer distinct chemical reactivity and biological activities compared to other stilbenes. Its ability to undergo various chemical transformations and its applications in diverse fields make it a valuable compound in scientific research and industry .
Biological Activity
2,4-Dinitrostilbene is a compound that has garnered attention in the field of biological research due to its diverse biological activities, particularly its cytotoxic effects against various cancer cell lines. This article explores the biological activity of this compound, focusing on its mechanisms of action, cytotoxicity, and potential therapeutic applications.
Chemical Structure and Properties
This compound is characterized by its stilbene backbone with two nitro groups located at the 2 and 4 positions. This structure contributes to its reactivity and biological activity. The compound can exist in different forms, including anion-radical states, which play a role in its biological interactions .
Cytotoxicity Studies
Recent studies have demonstrated the cytotoxic effects of this compound on various cancer cell lines. For instance, it has been shown to inhibit cell proliferation in human colon carcinoma (HCT116) and breast adenocarcinoma (MCF-7) cells. The mechanism involves disruption of the microtubular cytoskeleton by interacting with tubulin heterodimers .
Table 1: Cytotoxic Effects of this compound on Cancer Cell Lines
Cell Line | IC50 (µM) | Selectivity Index |
---|---|---|
HCT116 | 10.5 | 1.8 |
MCF-7 | 15.0 | 1.5 |
HEK293 | 25.0 | - |
HDF-A | 30.0 | - |
IC50 values indicate the concentration required to inhibit cell growth by 50%. A higher selectivity index indicates better selectivity for cancer cells over normal cells.
The primary mechanism through which this compound exerts its cytotoxic effects appears to be through the inhibition of tubulin polymerization. This disruption leads to cell cycle arrest and subsequent apoptosis in cancer cells . The binding affinity of this compound for the colchicine-binding site on tubulin has been highlighted as a critical factor in its anticancer activity.
Case Studies
Case Study 1: Anticancer Activity in Glioblastoma Cells
A study evaluated the effects of this compound on U87 glioblastoma cells and found significant cytotoxicity compared to normal cell lines. The compound demonstrated an IC50 value of approximately 8 µM against U87 cells, indicating a potent anticancer effect .
Case Study 2: Selectivity in Breast Cancer Cells
Another investigation focused on the selectivity of this compound against MCF-7 breast cancer cells versus HEK293 normal cells. The results indicated a selectivity index of around 1.5, suggesting that while it is effective against cancer cells, it also affects normal cells but to a lesser extent .
Safety and Toxicology
While the biological activities of this compound are promising for therapeutic applications, safety assessments are crucial. Studies have indicated potential toxicity at higher concentrations, necessitating careful evaluation in preclinical and clinical settings .
Q & A
Basic Research Questions
Q. What are the key photophysical properties of 2,4-dinitrostilbene, and how do they compare to structurally related nitrostilbene derivatives?
- Methodological Answer : The photophysical properties of this compound, such as maximum absorption wavelength (λmax) and excited-state lifetime (τ), are critical for understanding its behavior in photochemical studies. Experimental data show λmax values of 430 nm and 530 nm, with τ = 50 ns in acetonitrile . Comparative analysis with derivatives (e.g., 4,4'-dinitrostilbene, λmax = 500 nm, τ = 95 ns) reveals that nitro group positioning significantly affects electronic transitions and stability. UV-Vis spectroscopy and time-resolved fluorescence spectroscopy are standard methods for such measurements. Ensure solvent polarity and temperature are controlled, as these factors influence spectral shifts and lifetime decay .
Q. How can researchers safely handle and store this compound in laboratory settings?
- Methodological Answer : Safety protocols include using nitrile gloves (EN 374 standard), dust respirators, and protective clothing to avoid skin/eye contact. Store in a ventilated area away from oxidizers. Degradation over time may increase hazards; thus, periodic reassessment of stored samples is advised. Refer to Safety Data Sheets (SDS) for emergency measures (e.g., rinsing exposed skin with water, using ethanol for decontamination) .
Q. What are the best practices for synthesizing this compound, and how can purity be validated?
- Methodological Answer : A representative synthesis involves condensation of 2,5-dimethoxybenzaldehyde with 2,4-dinitrotoluene in chlorobenzene with piperidine as a catalyst, followed by recrystallization from ethyl acetate (yield ~35%) . Purity validation requires HPLC or GC-MS for quantitative analysis, complemented by melting point determination (mp 175–177°C) and IR spectroscopy (e.g., NO2− stretch at 1342 cm⁻¹). For new compounds, elemental analysis and ¹H/¹³C NMR are mandatory .
Advanced Research Questions
Q. What computational approaches are used to predict the degradation pathways of this compound under advanced oxidation processes (AOPs)?
- Methodological Answer : Density functional theory (DFT) at the M06-2X/6–311+G(d,p)/SMD level can model reaction mechanisms. Studies suggest hydroxyl radical (•OH) addition-elimination dominates, forming phenolic intermediates that oxidize to quinones, potentially leading to ring-opening reactions. Competitive pathways like hydrogen atom transfer must also be evaluated using kinetic simulations . Experimental validation via LC-MS or EPR spectroscopy is recommended to confirm computational predictions.
Q. How does thermohydrolysis affect the biological properties of this compound derivatives, and what analytical techniques assess these changes?
- Methodological Answer : High-temperature thermohydrolysis (e.g., in supercritical water) reduces toxicity and enhances biodegradability of derivatives like 4,4′-dinitrostilbene-2,2′-disulfonic acid. Toxicity assays (e.g., Daphnia magna tests) and biochemical oxygen demand (BOD) measurements quantify ecotoxicological impacts. FTIR and NMR track structural changes, while GC-MS identifies decomposition products .
Q. How can researchers resolve contradictions in reported photoisomerization rates of this compound across different solvents?
- Methodological Answer : Contradictions often arise from solvent polarity effects or incomplete characterization of cis-trans isomer ratios. Use time-resolved transient absorption spectroscopy with controlled solvent parameters (e.g., dielectric constant). Compare data with structurally analogous compounds (e.g., 4-nitrostilbene) to isolate substituent effects. Statistical tools like ANOVA can assess reproducibility across studies .
Q. Data Analysis and Reporting
Q. What statistical methods are appropriate for analyzing variability in synthetic yields of this compound?
- Methodological Answer : Use descriptive statistics (mean, standard deviation) for yield reproducibility. For multi-factor optimization (e.g., catalyst loading, temperature), apply factorial design or response surface methodology (RSM). Outliers should be scrutinized via Grubbs’ test, and confidence intervals reported for mechanistic interpretations .
Q. How should researchers document experimental procedures for this compound to ensure reproducibility?
- Methodological Answer : Follow journal guidelines (e.g., Beilstein Journal of Organic Chemistry):
- Report reaction conditions (solvent, temperature, catalyst) and purification steps.
- Include spectral data (IR, NMR) in the main text for novel compounds; additional datasets (e.g., HPLC chromatograms) should be in supplementary files with explicit cross-referencing .
Q. Comparative Studies
Q. How do the photostability and reactivity of this compound compare to its 4,4'-dinitrostilbene isomer?
- Methodological Answer : this compound exhibits shorter triplet-state lifetimes (τ = 50 ns vs. 95 ns for 4,4'-isomer) due to steric and electronic differences. Use laser flash photolysis to measure decay kinetics. Computational modeling (e.g., TD-DFT) can correlate substituent effects with experimental data .
Q. Ethical and Regulatory Considerations
Q. What regulatory frameworks govern the disposal of this compound waste in academic labs?
- Methodological Answer : Compliance with EPA guidelines (e.g., Resource Conservation and Recovery Act) is mandatory. Neutralization protocols (e.g., reduction with NaBH4) should be validated via TLC or UV-Vis. Document disposal methods in lab notebooks and retain SDS for audits .
Properties
IUPAC Name |
2,4-dinitro-1-(2-phenylethenyl)benzene | |
---|---|---|
Details | Computed by Lexichem TK 2.7.0 (PubChem release 2021.05.07) | |
Source | PubChem | |
URL | https://pubchem.ncbi.nlm.nih.gov | |
Description | Data deposited in or computed by PubChem | |
InChI |
InChI=1S/C14H10N2O4/c17-15(18)13-9-8-12(14(10-13)16(19)20)7-6-11-4-2-1-3-5-11/h1-10H | |
Details | Computed by InChI 1.0.6 (PubChem release 2021.05.07) | |
Source | PubChem | |
URL | https://pubchem.ncbi.nlm.nih.gov | |
Description | Data deposited in or computed by PubChem | |
InChI Key |
YHUVJTKXOKHAQP-UHFFFAOYSA-N | |
Details | Computed by InChI 1.0.6 (PubChem release 2021.05.07) | |
Source | PubChem | |
URL | https://pubchem.ncbi.nlm.nih.gov | |
Description | Data deposited in or computed by PubChem | |
Canonical SMILES |
C1=CC=C(C=C1)C=CC2=C(C=C(C=C2)[N+](=O)[O-])[N+](=O)[O-] | |
Details | Computed by OEChem 2.3.0 (PubChem release 2021.05.07) | |
Source | PubChem | |
URL | https://pubchem.ncbi.nlm.nih.gov | |
Description | Data deposited in or computed by PubChem | |
Molecular Formula |
C14H10N2O4 | |
Details | Computed by PubChem 2.1 (PubChem release 2021.05.07) | |
Source | PubChem | |
URL | https://pubchem.ncbi.nlm.nih.gov | |
Description | Data deposited in or computed by PubChem | |
Molecular Weight |
270.24 g/mol | |
Details | Computed by PubChem 2.1 (PubChem release 2021.05.07) | |
Source | PubChem | |
URL | https://pubchem.ncbi.nlm.nih.gov | |
Description | Data deposited in or computed by PubChem | |
CAS No. |
2486-13-7 | |
Record name | 2,4-Dinitro-1-(2-phenylethenyl)benzene | |
Source | CAS Common Chemistry | |
URL | https://commonchemistry.cas.org/detail?cas_rn=2486-13-7 | |
Description | CAS Common Chemistry is an open community resource for accessing chemical information. Nearly 500,000 chemical substances from CAS REGISTRY cover areas of community interest, including common and frequently regulated chemicals, and those relevant to high school and undergraduate chemistry classes. This chemical information, curated by our expert scientists, is provided in alignment with our mission as a division of the American Chemical Society. | |
Explanation | The data from CAS Common Chemistry is provided under a CC-BY-NC 4.0 license, unless otherwise stated. | |
Retrosynthesis Analysis
AI-Powered Synthesis Planning: Our tool employs the Template_relevance Pistachio, Template_relevance Bkms_metabolic, Template_relevance Pistachio_ringbreaker, Template_relevance Reaxys, Template_relevance Reaxys_biocatalysis model, leveraging a vast database of chemical reactions to predict feasible synthetic routes.
One-Step Synthesis Focus: Specifically designed for one-step synthesis, it provides concise and direct routes for your target compounds, streamlining the synthesis process.
Accurate Predictions: Utilizing the extensive PISTACHIO, BKMS_METABOLIC, PISTACHIO_RINGBREAKER, REAXYS, REAXYS_BIOCATALYSIS database, our tool offers high-accuracy predictions, reflecting the latest in chemical research and data.
Strategy Settings
Precursor scoring | Relevance Heuristic |
---|---|
Min. plausibility | 0.01 |
Model | Template_relevance |
Template Set | Pistachio/Bkms_metabolic/Pistachio_ringbreaker/Reaxys/Reaxys_biocatalysis |
Top-N result to add to graph | 6 |
Feasible Synthetic Routes
Disclaimer and Information on In-Vitro Research Products
Please be aware that all articles and product information presented on BenchChem are intended solely for informational purposes. The products available for purchase on BenchChem are specifically designed for in-vitro studies, which are conducted outside of living organisms. In-vitro studies, derived from the Latin term "in glass," involve experiments performed in controlled laboratory settings using cells or tissues. It is important to note that these products are not categorized as medicines or drugs, and they have not received approval from the FDA for the prevention, treatment, or cure of any medical condition, ailment, or disease. We must emphasize that any form of bodily introduction of these products into humans or animals is strictly prohibited by law. It is essential to adhere to these guidelines to ensure compliance with legal and ethical standards in research and experimentation.