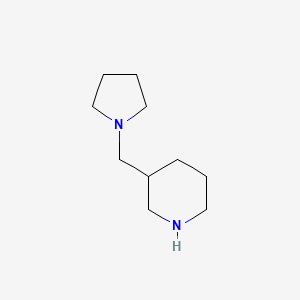
3-(Pyrrolidin-1-ylmethyl)piperidine
Overview
Description
3-(Pyrrolidin-1-ylmethyl)piperidine is a heterocyclic organic compound that features both pyrrolidine and piperidine rings. This compound is of significant interest in medicinal chemistry due to its potential biological activities and its role as a building block in the synthesis of various pharmacologically active molecules .
Mechanism of Action
Target of Action
The compound 3-(Pyrrolidin-1-ylmethyl)piperidine is a derivative of pyrrolidine and piperidine, two nitrogen-containing heterocycles widely used in medicinal chemistry . Pyrrolidine derivatives have been shown to target a variety of enzymes, including cholinesterase and beta secretase . Piperidine derivatives are also known to exhibit a wide range of biological activities .
Mode of Action
The pyrrolidine and piperidine moieties in the compound can contribute to its stereochemistry and increase its three-dimensional coverage due to the non-planarity of the rings . This allows the compound to efficiently explore the pharmacophore space and interact with its targets .
Biochemical Pathways
For instance, some pyrrolidine derivatives have demonstrated antiaggregatory and antioxidant effects .
Pharmacokinetics
The introduction of heteroatomic fragments in these molecules is known to modify physicochemical parameters and obtain the best adme/tox results for drug candidates .
Result of Action
Some pyrrolidine derivatives have been shown to exhibit both antiaggregatory and antioxidant effects .
Action Environment
The spatial orientation of substituents in pyrrolidine derivatives can lead to a different biological profile of drug candidates, due to the different binding mode to enantioselective proteins .
Preparation Methods
Synthetic Routes and Reaction Conditions: The synthesis of 3-(Pyrrolidin-1-ylmethyl)piperidine can be achieved through a multi-step process. One common method involves the exhaustive catalytic hydrogenation of pyrrolylpyridine. This process begins with the condensation of 3-aminopyridine with 2,5-dimethoxytetrahydrofuran to form pyrrolylpyridine. The pyrrolylpyridine is then subjected to catalytic hydrogenation in the presence of palladium on carbon and hydrochloric acid, resulting in the formation of this compound .
Industrial Production Methods: For industrial-scale production, the process can be scaled up using the same catalytic hydrogenation method. The use of palladium on carbon as a catalyst and the presence of hydrochloric acid ensure high yields and cost-effectiveness .
Chemical Reactions Analysis
Types of Reactions: 3-(Pyrrolidin-1-ylmethyl)piperidine undergoes various chemical reactions, including:
Oxidation: The compound can be oxidized using common oxidizing agents such as potassium permanganate or chromium trioxide.
Reduction: Reduction reactions can be carried out using hydrogen gas in the presence of a metal catalyst like palladium or platinum.
Substitution: Nucleophilic substitution reactions can occur at the nitrogen atoms of the pyrrolidine and piperidine rings.
Common Reagents and Conditions:
Oxidation: Potassium permanganate in an acidic medium.
Reduction: Hydrogen gas with palladium on carbon as a catalyst.
Substitution: Alkyl halides or acyl halides in the presence of a base.
Major Products:
Oxidation: Formation of N-oxides or other oxidized derivatives.
Reduction: Formation of fully saturated derivatives.
Substitution: Formation of N-alkyl or N-acyl derivatives.
Scientific Research Applications
3-(Pyrrolidin-1-ylmethyl)piperidine has a wide range of applications in scientific research:
Chemistry: Used as a building block in the synthesis of complex organic molecules.
Biology: Investigated for its potential biological activities, including antimicrobial and antiviral properties.
Medicine: Explored as a potential therapeutic agent for various diseases due to its ability to interact with biological targets.
Industry: Utilized in the production of pharmaceuticals and agrochemicals
Comparison with Similar Compounds
Pyrrolidine: A five-membered nitrogen-containing heterocycle.
Piperidine: A six-membered nitrogen-containing heterocycle.
N-Methylpiperidine: A methylated derivative of piperidine.
Uniqueness: 3-(Pyrrolidin-1-ylmethyl)piperidine is unique due to the presence of both pyrrolidine and piperidine rings in its structure. This dual-ring system provides a distinct three-dimensional conformation, enhancing its ability to interact with various biological targets and increasing its potential as a versatile scaffold in drug discovery .
Properties
IUPAC Name |
3-(pyrrolidin-1-ylmethyl)piperidine | |
---|---|---|
Source | PubChem | |
URL | https://pubchem.ncbi.nlm.nih.gov | |
Description | Data deposited in or computed by PubChem | |
InChI |
InChI=1S/C10H20N2/c1-2-7-12(6-1)9-10-4-3-5-11-8-10/h10-11H,1-9H2 | |
Source | PubChem | |
URL | https://pubchem.ncbi.nlm.nih.gov | |
Description | Data deposited in or computed by PubChem | |
InChI Key |
DJMGCHHOJIKYCP-UHFFFAOYSA-N | |
Source | PubChem | |
URL | https://pubchem.ncbi.nlm.nih.gov | |
Description | Data deposited in or computed by PubChem | |
Canonical SMILES |
C1CCN(C1)CC2CCCNC2 | |
Source | PubChem | |
URL | https://pubchem.ncbi.nlm.nih.gov | |
Description | Data deposited in or computed by PubChem | |
Molecular Formula |
C10H20N2 | |
Source | PubChem | |
URL | https://pubchem.ncbi.nlm.nih.gov | |
Description | Data deposited in or computed by PubChem | |
DSSTOX Substance ID |
DTXSID00402126 | |
Record name | 3-(pyrrolidin-1-ylmethyl)piperidine | |
Source | EPA DSSTox | |
URL | https://comptox.epa.gov/dashboard/DTXSID00402126 | |
Description | DSSTox provides a high quality public chemistry resource for supporting improved predictive toxicology. | |
Molecular Weight |
168.28 g/mol | |
Source | PubChem | |
URL | https://pubchem.ncbi.nlm.nih.gov | |
Description | Data deposited in or computed by PubChem | |
CAS No. |
514842-98-9 | |
Record name | 3-(pyrrolidin-1-ylmethyl)piperidine | |
Source | EPA DSSTox | |
URL | https://comptox.epa.gov/dashboard/DTXSID00402126 | |
Description | DSSTox provides a high quality public chemistry resource for supporting improved predictive toxicology. | |
Synthesis routes and methods
Procedure details
Disclaimer and Information on In-Vitro Research Products
Please be aware that all articles and product information presented on BenchChem are intended solely for informational purposes. The products available for purchase on BenchChem are specifically designed for in-vitro studies, which are conducted outside of living organisms. In-vitro studies, derived from the Latin term "in glass," involve experiments performed in controlled laboratory settings using cells or tissues. It is important to note that these products are not categorized as medicines or drugs, and they have not received approval from the FDA for the prevention, treatment, or cure of any medical condition, ailment, or disease. We must emphasize that any form of bodily introduction of these products into humans or animals is strictly prohibited by law. It is essential to adhere to these guidelines to ensure compliance with legal and ethical standards in research and experimentation.