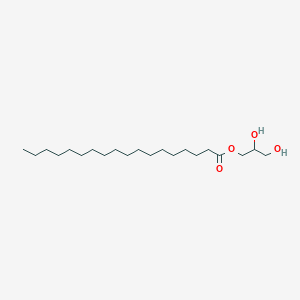
Glyceryl monostearate
Overview
Description
Glyceryl monostearate (GMS), chemically known as 2,3-dihydroxypropyl octadecanoate (C₂₁H₄₂O₄), is a monoglyceride derived from stearic acid and glycerol . It is widely used in pharmaceuticals, food, cosmetics, and plastics due to its emulsifying, stabilizing, and lipid-modifying properties. GMS exists as a white, waxy solid with a melting point of ~60–65°C and is approved by regulatory bodies such as the FDA, USP-NF, and Ph.Eur. for use in oral, topical, and industrial applications .
Preparation Methods
Chemical Synthesis via Esterification and Multistage Distillation
Reaction Mechanism and Stoichiometry
The industrial synthesis of GMS involves esterification of hydrogenated palm kernel oil (stearic acid source) with glycerol under alkaline catalysis. Sodium hydroxide (0.1–0.5 wt% relative to glycerol) facilitates proton exchange at 210–220°C, forming a crude GMS mixture containing 40–60% monoesters . The reaction follows second-order kinetics, with pressure maintained at -0.06 to -0.09 MPa to remove water byproduct and shift equilibrium toward ester formation .
Neutralization and Phase Separation
Post-esterification crude product undergoes phosphoric acid treatment (pH 6.0–7.5) to neutralize residual catalyst. This generates sodium phosphate precipitates, which are removed via 2-hour settling, achieving 98.5% dregs elimination . Filtration at 60–80°C prevents monoester recrystallization, yielding a semi-refined feedstock for distillation.
Four-Stage Vacuum Distillation Protocol
Purification employs sequential distillations under progressively stricter vacuum to separate glycerides by boiling point differences:
Stage | Temperature (°C) | Pressure (Pa) | Removed Components |
---|---|---|---|
1 | 160–180 | 150–180 | Moisture |
2 | 190–200 | 3–15 | Free glycerol |
3 | 200–210 | 3–8 | Diacylglycerols |
4 | 210–230 | 0.1–7 | Triacylglycerols |
Stage 4 distillates are spray-cooled to 25°C, producing GMS flakes with 99.1–99.2% monoester content, acid value ≤1.0 mg KOH/g, and iodine value ≤2.0 g I₂/100g .
Enzymatic Synthesis Using Immobilized Lipases
Candida antarctica Lipase B Catalysis
Novozym 435 (immobilized C. antarctica lipase B) catalyzes stearic acid-glycerol esterification in non-aqueous media. Optimal conditions include:
-
Substrate ratio : 1:3 stearic acid:glycerol (mol/mol), achieving 89% conversion in 2 hours
-
Solvent system : tert-butanol enhances glycerol solubility, creating monophasic reaction medium
-
Enzyme loading : 10% (w/w substrates) balances activity and cost
The lipase exhibits sn-1/3 regioselectivity, producing 95% α-monostearin and 5% β-isomer . Molecular sieves (3Å, 20% w/w) increase conversion by 12% through water adsorption .
Continuous Flow Reactor Performance
Packed-bed reactors (30 cm × 7.5 cm) with enzyme and molecular sieve layers operate at 2-hour residence time, maintaining 85% conversion over 15 cycles. Productivity reaches 0.48 g GMS/g enzyme/hr, outperforming batch systems by 22% .
Comparative Analysis of Synthesis Methods
Yield and Purity Metrics
Parameter | Chemical Method | Enzymatic Method |
---|---|---|
Monoester content | 99.2% | 89% |
Reaction time | 4.5 hours | 2 hours |
Energy consumption | 850 kWh/ton | 320 kWh/ton |
Catalyst cost | $12/ton | $150/ton |
Environmental and Regulatory Considerations
Chemical synthesis generates 50 kg phosphate sludge per ton of GMS, requiring wastewater treatment . Enzymatic routes reduce CO₂ emissions by 40% but face challenges in solvent recovery. The U.S. FDA classifies both methods as GRAS (21 CFR 184.1324), but EU regulations favor enzymatic GMS in organic products (EC 834/2007).
Quality Control and Characterization
Key Analytical Parameters
-
FT-IR verification : C=O stretch at 1735 cm⁻¹, OH stretch at 3470 cm⁻¹
HPLC Quantification Protocol
Reverse-phase C18 columns (250 × 4.6 mm, 5 μm) with UV detection at 205 nm resolve mono-, di-, and triacylglycerols. Mobile phase: acetonitrile/isopropanol (70:30) at 1.0 mL/min. Retention times: GMS (8.2 min), distearin (12.7 min), stearic acid (4.5 min) .
Industrial Scale-Up Challenges
Distillation Column Fouling
High-melt (70°C) GMS causes fouling in stage 4 evaporators. Patent CN102964245A addresses this through:
-
Thermal oil heating (0.25–0.3 MPa pressure) maintaining 5°C superheat
-
Short-path distillation with rotor-scraped surfaces
Enzyme Deactivation Mechanisms
Lipase activity drops 15% per cycle due to:
Chemical Reactions Analysis
Types of Reactions: Glyceryl monostearate primarily undergoes esterification and hydrolysis reactions. It can also participate in transesterification reactions when mixed with other esters or alcohols .
Common Reagents and Conditions:
Esterification: Stearic acid and glycerol, with an esterification catalyst.
Hydrolysis: Water and a hydrolysis catalyst under reflux conditions.
Transesterification: Other esters or alcohols in the presence of a catalyst.
Major Products Formed:
Esterification: this compound.
Hydrolysis: Glycerol and stearic acid.
Transesterification: Various mono-, di-, and triglycerides.
Scientific Research Applications
Pharmaceutical Applications
Glyceryl monostearate is primarily utilized in drug delivery systems due to its biocompatibility and ability to enhance the solubility of poorly water-soluble drugs.
Drug Delivery Systems
- Thermal-Sensitive Drug Delivery: GMS has been incorporated into lipid matrices for developing magnetically induced thermo-responsive drug delivery systems. For instance, a study evaluated a blend of glyceryl monooleate and GMS for delivering nifedipine, demonstrating temperature-dependent phase transitions suitable for controlled drug release .
- Sustained Release Formulations: Research has focused on designing implantable systems using GMS for localized antibiotic delivery. The release kinetics of cefazolin from GMS matrices were analyzed using agar gel models, indicating effective spatial and temporal drug release profiles .
- Solid Lipid Nanoparticles (SLN): GMS is a key component in the formulation of SLNs. A study reported the successful incorporation of tretinoin into SLNs fabricated from biocompatible microemulsions containing GMS, achieving an encapsulation efficiency of 37-48% .
Ocular Drug Delivery
GMS has been explored for ocular applications due to its ability to improve drug retention in the eye. Research indicates that lipophilic prodrugs of antiviral agents can be effectively delivered using GMS-based formulations, enhancing therapeutic concentrations in ocular tissues .
Food Technology Applications
In food science, GMS serves as an emulsifier and stabilizer, improving texture and shelf-life.
Emulsification Properties
GMS enhances the stability of emulsions in food products, which is critical in preventing separation and maintaining quality. Its ability to interact with both oil and water phases makes it effective in various formulations.
Bioavailability Studies
Recent studies have examined how GMS affects the bioavailability of contaminants like phthalate esters in food products. One study found that GMS increased internal exposure levels of these contaminants in male rats, raising concerns about its safety as a food additive .
Cosmetic Applications
In cosmetics, GMS is used for its emulsifying properties, contributing to the stability and texture of creams and lotions.
Skin Care Formulations
GMS is commonly included in skin care products as an emulsifier that helps blend oil and water components, providing a smooth application and enhancing product stability.
Comparative Studies
A comparative study between GMS and beeswax-based oleogels showed that GMS-based formulations exhibited superior firmness and structural integrity, highlighting its potential in creating stable gel-like textures for various applications .
Data Tables
Here are some summarized findings from various studies:
Case Studies
- Thermal-Sensitive Drug Delivery System: A study demonstrated the use of a GMS-based matrix for controlled release under thermal conditions, showing significant potential for localized chemotherapy applications .
- Ocular Drug Delivery: Research on lipophilic prodrugs utilizing GMS showed enhanced retention times for antiviral agents in ocular tissues, suggesting its effectiveness in treating eye diseases .
Mechanism of Action
Glyceryl monostearate functions primarily as an emulsifier due to its amphiphilic structure, which contains both hydrophilic and lipophilic regions. This structure allows it to reduce the surface tension between immiscible liquids, such as oil and water, thereby stabilizing emulsions . In pharmaceutical applications, it helps control the release of active ingredients by forming a barrier that modulates drug diffusion .
Comparison with Similar Compounds
Thermal and Structural Properties
GMS exhibits distinct thermal behaviors compared to other glyceryl esters and lipids:
Key Findings :
- GMS’s lower melting point (~60–65°C) compared to glyceryl behenate (77.47°C) or trimyristate (74.53°C) makes it suitable for melt granulation and lipid nanoparticles .
- GMS enhances crystallinity and thermal stability in oleogels compared to glyceryl monolaurate, improving mechanical strength .
Drug Delivery Systems
- Solid Lipid Nanoparticles (SLNs): GMS forms stable SLNs with entrapment efficiencies comparable to glyceryl trimyristate but requires surfactants for microemulsion formation. Glyceryl behenate-based SLNs show slower drug release due to higher melting points .
- Sustained-Release Matrices : GMS retards drug release more effectively than beeswax in melt granulation. Combining GMS with stearic acid further prolongs release .
Food and Cosmetics
- Oleogels: GMS produces denser 3D networks than glyceryl monolaurate, enhancing deformation resistance in margarines and shortenings .
- Emulsification: Self-emulsifying GMS grades outperform monooleate in stabilizing oil-in-water creams, reducing phase separation .
Crystallization and Polymorphism
GMS modifies crystallization dynamics in lipid systems:
- At 1–4 wt%, GMS increases crystallization temperature in palm stearin and promotes β'-form crystals, which resist enzymatic breakdown in starch matrices .
- In contrast, sorbitan monopalmitate favors β-form crystals, which are less desirable in food textures .
Regulatory and Compositional Variability
- Regulatory Status: GMS, glyceryl monooleate, and glyceryl behenate are FDA-approved, but GMS is unique in its GRAS status for food and topical use .
- Composition: Commercial GMS contains 40–55% monoglycerides, with variability in palmitate/oleate content, unlike purer derivatives like glyceryl behenate (≥85% monoglycerides) .
Biological Activity
Glyceryl monostearate (GMS), a nonionic surfactant and emulsifier derived from glycerol and stearic acid, exhibits a variety of biological activities that make it valuable in pharmaceutical, cosmetic, and food industries. This article explores its synthesis, physicochemical properties, and biological applications, supported by data tables and relevant case studies.
Synthesis of this compound
GMS can be synthesized through enzymatic processes, particularly using lipases such as Novozym 435 and immobilized Candida antarctica B lipase. These methods provide a more selective approach compared to traditional chemical synthesis, allowing for the production of high-purity monoglycerides.
Key Parameters in Synthesis:
- Molar Ratio of Substrates: A study revealed that increasing the glycerol concentration in the reaction mixture enhanced the conversion rate of stearic acid to GMS. Optimal conditions were found at a 1:3 molar ratio of stearic acid to glycerol, achieving approximately 82% conversion within 2 hours at 60°C .
- Enzyme Efficiency: The efficiency of different lipases was compared, with immobilized Candida antarctica B lipase showing competitive conversion rates comparable to Novozym 435 .
GMS is amphiphilic, allowing it to self-assemble into micelles in aqueous solutions. Its critical micelle concentration (CMC) is approximately 30 µmol/L at room temperature, which is significant for its applications in drug delivery systems . The melting and crystallization points of GMS are crucial for its use in thermal-sensitive drug delivery systems, with blends showing phase transitions at specific temperatures that can be exploited for controlled drug release .
Drug Delivery Systems
GMS has been evaluated for its role in various drug delivery systems due to its biocompatibility and ability to enhance drug solubility.
- Thermo-responsive Drug Delivery: A study investigated a GMS-based matrix combined with glyceryl monooleate for local chemotherapy applications. The matrix demonstrated temperature-dependent phase transitions suitable for on-demand drug release .
- Solid Lipid Nanoparticles (SLNs): GMS has been used to formulate SLNs for improved bioavailability of drugs like apomorphine in Parkinson's disease models. These SLNs showed significantly higher bioavailability compared to traditional formulations .
Case Studies
- Sustained Release Coating Formulations: Research on sustained release coatings using GMS highlighted its effectiveness in maintaining drug release profiles over extended periods when implanted in simulated biological environments .
- Antibiotic Delivery Systems: GMS matrices were developed for the localized delivery of antibiotics such as cefazolin, demonstrating controlled release characteristics that could prevent surgical site infections .
Summary of Findings
Parameter | Value/Observation |
---|---|
CMC (Critical Micelle Concentration) | 30 µmol/L |
Optimal Molar Ratio (Stearic Acid:Glycerol) | 1:3 |
Conversion Rate | ~82% in 2 hours |
Melting Point | 42°C |
Crystallization Point | 37°C |
Q & A
Basic Research Questions
Q. How can researchers determine monoglyceride content and free glycerol in GMS to ensure batch consistency?
- Methodological Answer : Use reversed-phase HPLC with a C18 column and a refractive index detector. Prepare a calibration curve using glycerol standards (0.4–4.0 mg/mL) to quantify free glycerol . For monoglyceride content, employ size-exclusion chromatography (SEC) to separate mono-, di-, and triacylglycerols, comparing retention times against pharmacopeial reference standards (e.g., USP Monoglycerides RS) . Free glycerol should not exceed 6.0% (w/w), as per USP/Ph.Eur. guidelines .
Q. What experimental design strategies optimize GMS-based formulations for drug delivery systems?
- Methodological Answer : Apply a Design of Experiments (DoE) approach, such as Box-Behnken or factorial design, to evaluate critical factors like lipid concentration, surfactant ratio, and processing temperature. For solid lipid nanoparticles (SLNs), optimize particle size and entrapment efficiency by varying GMS content (e.g., 5–15% w/w) and homogenization cycles . Use response surface models to predict optimal conditions and validate with in vitro release studies .
Q. How do pharmacopeial standards (e.g., USP, Ph.Eur.) guide GMS characterization for research use?
- Methodological Answer : Follow monographs specifying fatty acid composition (≥90% saturated monoacylglycerols), heavy metal limits (≤1 ppm nickel), and water content (≤1.0%) . For Type I/II/III grades, verify iodine value (≤3) and saponification value (145–165) to distinguish between stearate/palmitate ratios . Include labeling of antioxidants (e.g., BHT) if added during synthesis .
Q. What role does GMS play in controlled-release formulations?
- Methodological Answer : Leverage the β-polymorph of GMS, which forms stable crystalline matrices for sustained drug release. Prepare matrices by melt granulation (60–70°C) and characterize using differential scanning calorimetry (DSC) to confirm polymorphic transitions (α → β at ~55°C). In vitro dissolution studies in pH 6.8 buffers can demonstrate prolonged release over 12–24 hours .
Advanced Research Questions
Q. How do synthesis methods (e.g., glycerolysis vs. stearoyl chloride reaction) affect GMS physicochemical properties?
- Methodological Answer : Compare glycerolysis-derived GMS (from triglycerides) with stearoyl chloride-synthesized batches. Analyze fatty acid profiles via GC-MS to detect palmitate/oleate impurities. Glycerolysis yields 40–55% monoacylglycerols, requiring distillation for ≥90% purity, while stearoyl chloride methods introduce HCl byproducts requiring neutralization . Assess melting points (54–66°C) and emulsification efficiency to correlate synthesis with functionality .
Q. What advanced techniques characterize GMS polymorphic transitions in lipid-based systems?
- Methodological Answer : Use synchrotron X-ray diffraction (XRD) to identify α (hexagonal, metastable) and β (triclinic, stable) polymorphs. Couple with atomic force microscopy (AFM) to study crystal growth kinetics during cooling (e.g., 5°C/min). Polymorphic stability impacts emulsification (α-form) vs. matrix rigidity (β-form) in SLNs .
Q. How do GMS-surfactant interactions influence colloidal stability in lipid nanoparticles?
- Methodological Answer : Screen surfactants (e.g., Poloxamer 188, Tween 80) using surface tension measurements to identify synergistic effects. For SLNs, stabilize oil-water interfaces by combining GMS (3% w/w) with lecithin (1.5% w/w). Monitor particle aggregation via dynamic light scattering (DLS) over 30 days at 25°C .
Q. What strategies resolve contradictions in GMS emulsification efficiency reported across studies?
- Methodological Answer : Conduct systematic reviews to identify variables like purity (≥90% vs. 40–55% monoacylglycerols), fatty acid chain length, and surfactant co-formulations. Validate through meta-analysis of HLB values: GMS (HLB 3–4) requires hydrophilic surfactants (HLB >12) for oil-in-water emulsions .
Q. What are the critical stability challenges when incorporating GMS into lipid nanoparticles?
- Methodological Answer : Address hydrolysis by storing SLNs at 4°C in amber vials to minimize UV/heat exposure. Quantify free fatty acids via titration (acid value ≤2 mg KOH/g) and monitor polymorphic transitions using hot-stage microscopy. Lyophilize with cryoprotectants (e.g., trehalose 5% w/v) to prevent particle fusion .
Properties
IUPAC Name |
2,3-dihydroxypropyl octadecanoate | |
---|---|---|
Source | PubChem | |
URL | https://pubchem.ncbi.nlm.nih.gov | |
Description | Data deposited in or computed by PubChem | |
InChI |
InChI=1S/C21H42O4/c1-2-3-4-5-6-7-8-9-10-11-12-13-14-15-16-17-21(24)25-19-20(23)18-22/h20,22-23H,2-19H2,1H3 | |
Source | PubChem | |
URL | https://pubchem.ncbi.nlm.nih.gov | |
Description | Data deposited in or computed by PubChem | |
InChI Key |
VBICKXHEKHSIBG-UHFFFAOYSA-N | |
Source | PubChem | |
URL | https://pubchem.ncbi.nlm.nih.gov | |
Description | Data deposited in or computed by PubChem | |
Canonical SMILES |
CCCCCCCCCCCCCCCCCC(=O)OCC(CO)O | |
Source | PubChem | |
URL | https://pubchem.ncbi.nlm.nih.gov | |
Description | Data deposited in or computed by PubChem | |
Molecular Formula |
C21H42O4 | |
Source | PubChem | |
URL | https://pubchem.ncbi.nlm.nih.gov | |
Description | Data deposited in or computed by PubChem | |
DSSTOX Substance ID |
DTXSID7029160 | |
Record name | Glycerol 1-monostearate | |
Source | EPA DSSTox | |
URL | https://comptox.epa.gov/dashboard/DTXSID7029160 | |
Description | DSSTox provides a high quality public chemistry resource for supporting improved predictive toxicology. | |
Molecular Weight |
358.6 g/mol | |
Source | PubChem | |
URL | https://pubchem.ncbi.nlm.nih.gov | |
Description | Data deposited in or computed by PubChem | |
Physical Description |
White waxy solid; [Merck Index], white to pale yellow wax-like solid with a mild fatty odour | |
Record name | Glyceryl monostearate | |
Source | Haz-Map, Information on Hazardous Chemicals and Occupational Diseases | |
URL | https://haz-map.com/Agents/14436 | |
Description | Haz-Map® is an occupational health database designed for health and safety professionals and for consumers seeking information about the adverse effects of workplace exposures to chemical and biological agents. | |
Explanation | Copyright (c) 2022 Haz-Map(R). All rights reserved. Unless otherwise indicated, all materials from Haz-Map are copyrighted by Haz-Map(R). No part of these materials, either text or image may be used for any purpose other than for personal use. Therefore, reproduction, modification, storage in a retrieval system or retransmission, in any form or by any means, electronic, mechanical or otherwise, for reasons other than personal use, is strictly prohibited without prior written permission. | |
Record name | Glyceryl monostearate | |
Source | Joint FAO/WHO Expert Committee on Food Additives (JECFA) | |
URL | https://www.fao.org/food/food-safety-quality/scientific-advice/jecfa/jecfa-flav/details/en/c/778/ | |
Description | The flavoring agent databse provides the most recent specifications for flavorings evaluated by JECFA. | |
Explanation | Permission from WHO is not required for the use of WHO materials issued under the Creative Commons Attribution-NonCommercial-ShareAlike 3.0 Intergovernmental Organization (CC BY-NC-SA 3.0 IGO) licence. | |
Solubility |
insoluble in water; soluble in hot oils, organic solvents, soluble in hot alcohol (in ethanol) | |
Record name | Glyceryl monostearate | |
Source | Joint FAO/WHO Expert Committee on Food Additives (JECFA) | |
URL | https://www.fao.org/food/food-safety-quality/scientific-advice/jecfa/jecfa-flav/details/en/c/778/ | |
Description | The flavoring agent databse provides the most recent specifications for flavorings evaluated by JECFA. | |
Explanation | Permission from WHO is not required for the use of WHO materials issued under the Creative Commons Attribution-NonCommercial-ShareAlike 3.0 Intergovernmental Organization (CC BY-NC-SA 3.0 IGO) licence. | |
CAS No. |
123-94-4, 14811-92-8, 31566-31-1, 83138-62-9 | |
Record name | 1-Monostearin | |
Source | CAS Common Chemistry | |
URL | https://commonchemistry.cas.org/detail?cas_rn=123-94-4 | |
Description | CAS Common Chemistry is an open community resource for accessing chemical information. Nearly 500,000 chemical substances from CAS REGISTRY cover areas of community interest, including common and frequently regulated chemicals, and those relevant to high school and undergraduate chemistry classes. This chemical information, curated by our expert scientists, is provided in alignment with our mission as a division of the American Chemical Society. | |
Explanation | The data from CAS Common Chemistry is provided under a CC-BY-NC 4.0 license, unless otherwise stated. | |
Record name | Glyceryl monostearate | |
Source | ChemIDplus | |
URL | https://pubchem.ncbi.nlm.nih.gov/substance/?source=chemidplus&sourceid=0000123944 | |
Description | ChemIDplus is a free, web search system that provides access to the structure and nomenclature authority files used for the identification of chemical substances cited in National Library of Medicine (NLM) databases, including the TOXNET system. | |
Record name | (R)-2,3-Dihydroxypropyl stearate | |
Source | ChemIDplus | |
URL | https://pubchem.ncbi.nlm.nih.gov/substance/?source=chemidplus&sourceid=0014811928 | |
Description | ChemIDplus is a free, web search system that provides access to the structure and nomenclature authority files used for the identification of chemical substances cited in National Library of Medicine (NLM) databases, including the TOXNET system. | |
Record name | Glyceryl monostearate [JAN:NF] | |
Source | ChemIDplus | |
URL | https://pubchem.ncbi.nlm.nih.gov/substance/?source=chemidplus&sourceid=0031566311 | |
Description | ChemIDplus is a free, web search system that provides access to the structure and nomenclature authority files used for the identification of chemical substances cited in National Library of Medicine (NLM) databases, including the TOXNET system. | |
Record name | 1,2,3-Propanetriol, homopolymer, isooctadecanoate | |
Source | ChemIDplus | |
URL | https://pubchem.ncbi.nlm.nih.gov/substance/?source=chemidplus&sourceid=0083138629 | |
Description | ChemIDplus is a free, web search system that provides access to the structure and nomenclature authority files used for the identification of chemical substances cited in National Library of Medicine (NLM) databases, including the TOXNET system. | |
Record name | Glyceryl monostearate | |
Source | DrugBank | |
URL | https://www.drugbank.ca/drugs/DB11250 | |
Description | The DrugBank database is a unique bioinformatics and cheminformatics resource that combines detailed drug (i.e. chemical, pharmacological and pharmaceutical) data with comprehensive drug target (i.e. sequence, structure, and pathway) information. | |
Explanation | Creative Common's Attribution-NonCommercial 4.0 International License (http://creativecommons.org/licenses/by-nc/4.0/legalcode) | |
Record name | Tegin | |
Source | DTP/NCI | |
URL | https://dtp.cancer.gov/dtpstandard/servlet/dwindex?searchtype=NSC&outputformat=html&searchlist=3875 | |
Description | The NCI Development Therapeutics Program (DTP) provides services and resources to the academic and private-sector research communities worldwide to facilitate the discovery and development of new cancer therapeutic agents. | |
Explanation | Unless otherwise indicated, all text within NCI products is free of copyright and may be reused without our permission. Credit the National Cancer Institute as the source. | |
Record name | Glycerol 1-monostearate | |
Source | EPA DSSTox | |
URL | https://comptox.epa.gov/dashboard/DTXSID7029160 | |
Description | DSSTox provides a high quality public chemistry resource for supporting improved predictive toxicology. | |
Record name | Stearic acid, monoester with glycerol | |
Source | European Chemicals Agency (ECHA) | |
URL | https://echa.europa.eu/substance-information/-/substanceinfo/100.046.081 | |
Description | The European Chemicals Agency (ECHA) is an agency of the European Union which is the driving force among regulatory authorities in implementing the EU's groundbreaking chemicals legislation for the benefit of human health and the environment as well as for innovation and competitiveness. | |
Explanation | Use of the information, documents and data from the ECHA website is subject to the terms and conditions of this Legal Notice, and subject to other binding limitations provided for under applicable law, the information, documents and data made available on the ECHA website may be reproduced, distributed and/or used, totally or in part, for non-commercial purposes provided that ECHA is acknowledged as the source: "Source: European Chemicals Agency, http://echa.europa.eu/". Such acknowledgement must be included in each copy of the material. ECHA permits and encourages organisations and individuals to create links to the ECHA website under the following cumulative conditions: Links can only be made to webpages that provide a link to the Legal Notice page. | |
Record name | GLYCERYL 1-STEARATE | |
Source | FDA Global Substance Registration System (GSRS) | |
URL | https://gsrs.ncats.nih.gov/ginas/app/beta/substances/258491E1RZ | |
Description | The FDA Global Substance Registration System (GSRS) enables the efficient and accurate exchange of information on what substances are in regulated products. Instead of relying on names, which vary across regulatory domains, countries, and regions, the GSRS knowledge base makes it possible for substances to be defined by standardized, scientific descriptions. | |
Explanation | Unless otherwise noted, the contents of the FDA website (www.fda.gov), both text and graphics, are not copyrighted. They are in the public domain and may be republished, reprinted and otherwise used freely by anyone without the need to obtain permission from FDA. Credit to the U.S. Food and Drug Administration as the source is appreciated but not required. | |
Synthesis routes and methods I
Procedure details
Synthesis routes and methods II
Procedure details
Synthesis routes and methods III
Procedure details
Retrosynthesis Analysis
AI-Powered Synthesis Planning: Our tool employs the Template_relevance Pistachio, Template_relevance Bkms_metabolic, Template_relevance Pistachio_ringbreaker, Template_relevance Reaxys, Template_relevance Reaxys_biocatalysis model, leveraging a vast database of chemical reactions to predict feasible synthetic routes.
One-Step Synthesis Focus: Specifically designed for one-step synthesis, it provides concise and direct routes for your target compounds, streamlining the synthesis process.
Accurate Predictions: Utilizing the extensive PISTACHIO, BKMS_METABOLIC, PISTACHIO_RINGBREAKER, REAXYS, REAXYS_BIOCATALYSIS database, our tool offers high-accuracy predictions, reflecting the latest in chemical research and data.
Strategy Settings
Precursor scoring | Relevance Heuristic |
---|---|
Min. plausibility | 0.01 |
Model | Template_relevance |
Template Set | Pistachio/Bkms_metabolic/Pistachio_ringbreaker/Reaxys/Reaxys_biocatalysis |
Top-N result to add to graph | 6 |
Feasible Synthetic Routes
Disclaimer and Information on In-Vitro Research Products
Please be aware that all articles and product information presented on BenchChem are intended solely for informational purposes. The products available for purchase on BenchChem are specifically designed for in-vitro studies, which are conducted outside of living organisms. In-vitro studies, derived from the Latin term "in glass," involve experiments performed in controlled laboratory settings using cells or tissues. It is important to note that these products are not categorized as medicines or drugs, and they have not received approval from the FDA for the prevention, treatment, or cure of any medical condition, ailment, or disease. We must emphasize that any form of bodily introduction of these products into humans or animals is strictly prohibited by law. It is essential to adhere to these guidelines to ensure compliance with legal and ethical standards in research and experimentation.