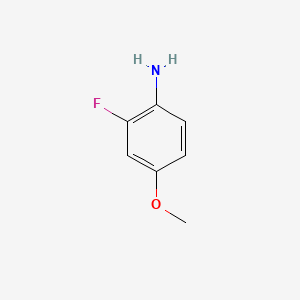
2-Fluoro-4-methoxyaniline
Overview
Description
2-Fluoro-4-methoxyaniline is a chemical compound with the molecular formula C7H8FNO. It has a molecular weight of 141.15 . It is a pale-yellow or light-green to brown or pale-gray solid or liquid .
Molecular Structure Analysis
The molecular structure of 2-Fluoro-4-methoxyaniline consists of a benzene ring substituted with a fluoro group at the 2nd position and a methoxy group at the 4th position . The compound also has an amine group attached to the benzene ring .Physical And Chemical Properties Analysis
2-Fluoro-4-methoxyaniline has a density of 1.2±0.1 g/cm3, a boiling point of 203.0±20.0 °C at 760 mmHg, and a vapor pressure of 0.3±0.4 mmHg at 25°C . It also has a molar refractivity of 37.2±0.3 cm3, a polar surface area of 35 Å2, and a molar volume of 119.9±3.0 cm3 .Scientific Research Applications
Leimgruber-Batcho Reaction
This compound is used in the preparation of 6-chloro-5-fluoroindole through the Leimgruber-Batcho reaction, which is significant in synthesizing indole derivatives .
Synthesis of Amino Alcohols
It is also involved in synthesizing an (S)-amino alcohol, 2-amino-3-(2-fluoro-4-methylphenyl)-propan-1-ol, which can have various applications in chemical synthesis and pharmaceuticals .
Chemical Synthesis Research
Sigma-Aldrich provides 2-Fluoro-4-methoxyaniline to early discovery researchers as part of a collection of unique chemicals for use in chemical synthesis research .
Material Science Applications
Scientists with experience in material science may utilize this compound in developing new materials with specific desired properties .
Mechanism of Action
Target of Action
2-Fluoro-4-methoxyaniline is a unique chemical compound that is used in early discovery research . It is a key building block in the synthesis of osimertinib , a third-generation epidermal growth factor receptor tyrosine kinase inhibitor (EGFR-TKI) that is used for the treatment of non-small-cell lung carcinomas carrying EGFR-TKI sensitizing and EGFR T790M resistance mutations .
Mode of Action
It is known to undergo nitration, a process that is predominantly kinetically controlled . This suggests that the compound interacts with its targets through chemical reactions, leading to changes in the molecular structure of the targets.
Biochemical Pathways
Given its role in the synthesis of osimertinib, it can be inferred that it may be involved in pathways related to the inhibition of egfr-tki, which plays a crucial role in the proliferation of non-small-cell lung carcinomas .
Pharmacokinetics
It is known that the compound has a high gastrointestinal absorption and is bbb permeant . Its log Kp (skin permeation) is -6.16 cm/s . These properties suggest that the compound has good bioavailability.
Result of Action
Given its role in the synthesis of osimertinib, it can be inferred that its action may result in the inhibition of egfr-tki, thereby affecting the proliferation of non-small-cell lung carcinomas .
Action Environment
The action of 2-Fluoro-4-methoxyaniline can be influenced by various environmental factors. For instance, its nitration process is temperature-dependent, with optimal results achieved at 70°C . Additionally, its solubility, which can affect its bioavailability and efficacy, is influenced by the solvent used .
Safety and Hazards
2-Fluoro-4-methoxyaniline is considered hazardous. It has a GHS07 pictogram and a warning signal word . The hazard statements include H302, H315, H319, H332, H335, indicating that it is harmful if swallowed, causes skin irritation, causes serious eye irritation, harmful if inhaled, and may cause respiratory irritation .
properties
IUPAC Name |
2-fluoro-4-methoxyaniline | |
---|---|---|
Source | PubChem | |
URL | https://pubchem.ncbi.nlm.nih.gov | |
Description | Data deposited in or computed by PubChem | |
InChI |
InChI=1S/C7H8FNO/c1-10-5-2-3-7(9)6(8)4-5/h2-4H,9H2,1H3 | |
Source | PubChem | |
URL | https://pubchem.ncbi.nlm.nih.gov | |
Description | Data deposited in or computed by PubChem | |
InChI Key |
YWUVOJJHVFLNJA-UHFFFAOYSA-N | |
Source | PubChem | |
URL | https://pubchem.ncbi.nlm.nih.gov | |
Description | Data deposited in or computed by PubChem | |
Canonical SMILES |
COC1=CC(=C(C=C1)N)F | |
Source | PubChem | |
URL | https://pubchem.ncbi.nlm.nih.gov | |
Description | Data deposited in or computed by PubChem | |
Molecular Formula |
C7H8FNO | |
Source | PubChem | |
URL | https://pubchem.ncbi.nlm.nih.gov | |
Description | Data deposited in or computed by PubChem | |
DSSTOX Substance ID |
DTXSID60395989 | |
Record name | 2-fluoro-4-methoxyaniline | |
Source | EPA DSSTox | |
URL | https://comptox.epa.gov/dashboard/DTXSID60395989 | |
Description | DSSTox provides a high quality public chemistry resource for supporting improved predictive toxicology. | |
Molecular Weight |
141.14 g/mol | |
Source | PubChem | |
URL | https://pubchem.ncbi.nlm.nih.gov | |
Description | Data deposited in or computed by PubChem | |
Product Name |
2-Fluoro-4-methoxyaniline | |
CAS RN |
458-52-6 | |
Record name | 2-fluoro-4-methoxyaniline | |
Source | EPA DSSTox | |
URL | https://comptox.epa.gov/dashboard/DTXSID60395989 | |
Description | DSSTox provides a high quality public chemistry resource for supporting improved predictive toxicology. | |
Synthesis routes and methods I
Procedure details
Synthesis routes and methods II
Procedure details
Synthesis routes and methods III
Procedure details
Synthesis routes and methods IV
Procedure details
Synthesis routes and methods V
Procedure details
Retrosynthesis Analysis
AI-Powered Synthesis Planning: Our tool employs the Template_relevance Pistachio, Template_relevance Bkms_metabolic, Template_relevance Pistachio_ringbreaker, Template_relevance Reaxys, Template_relevance Reaxys_biocatalysis model, leveraging a vast database of chemical reactions to predict feasible synthetic routes.
One-Step Synthesis Focus: Specifically designed for one-step synthesis, it provides concise and direct routes for your target compounds, streamlining the synthesis process.
Accurate Predictions: Utilizing the extensive PISTACHIO, BKMS_METABOLIC, PISTACHIO_RINGBREAKER, REAXYS, REAXYS_BIOCATALYSIS database, our tool offers high-accuracy predictions, reflecting the latest in chemical research and data.
Strategy Settings
Precursor scoring | Relevance Heuristic |
---|---|
Min. plausibility | 0.01 |
Model | Template_relevance |
Template Set | Pistachio/Bkms_metabolic/Pistachio_ringbreaker/Reaxys/Reaxys_biocatalysis |
Top-N result to add to graph | 6 |
Feasible Synthetic Routes
Q & A
Q1: What are the main synthetic challenges in producing 2-fluoro-4-methoxyaniline?
A1: One of the main challenges in synthesizing 2-fluoro-4-methoxyaniline lies in achieving regioselectivity during the nitration of aromatic rings. [] Traditional methods often lead to a mixture of isomers, requiring tedious purification steps. To address this, researchers have developed alternative approaches, such as the Ullman route, which avoids non-selective nitration reactions and offers a more controlled synthesis of the desired 2-fluoro-4-methoxyaniline isomer. []
Q2: Can you describe an alternative synthetic route for 2-fluoro-4-methoxyaniline that avoids non-selective nitration?
A2: Yes, an alternative route involves the use of the Ullman reaction. [] This method utilizes readily available starting materials like 2-fluoro-4-iodoaniline and copper(I) iodide as a catalyst to introduce the methoxy group selectively at the desired position. The reaction proceeds through a copper-mediated coupling with methanol, leading to the formation of 2-fluoro-4-methoxyaniline with high regioselectivity. This approach avoids the challenges associated with non-selective nitration, offering a more efficient and controlled synthesis. []
Q3: Apart from its role as a synthetic intermediate, are there any documented applications of 2-fluoro-4-methoxyaniline?
A3: While the provided research primarily highlights the use of 2-fluoro-4-methoxyaniline as a key building block in the multi-step synthesis of complex molecules, its applications extend beyond that. [, ] It can serve as a versatile precursor for various chemical transformations, enabling the introduction of the 2-fluoro-4-methoxyphenyl moiety into diverse molecular scaffolds. This opens up possibilities for exploring its potential in areas like medicinal chemistry, material science, and agrochemical development. Further research is necessary to fully elucidate its properties and explore a broader range of applications.
Disclaimer and Information on In-Vitro Research Products
Please be aware that all articles and product information presented on BenchChem are intended solely for informational purposes. The products available for purchase on BenchChem are specifically designed for in-vitro studies, which are conducted outside of living organisms. In-vitro studies, derived from the Latin term "in glass," involve experiments performed in controlled laboratory settings using cells or tissues. It is important to note that these products are not categorized as medicines or drugs, and they have not received approval from the FDA for the prevention, treatment, or cure of any medical condition, ailment, or disease. We must emphasize that any form of bodily introduction of these products into humans or animals is strictly prohibited by law. It is essential to adhere to these guidelines to ensure compliance with legal and ethical standards in research and experimentation.