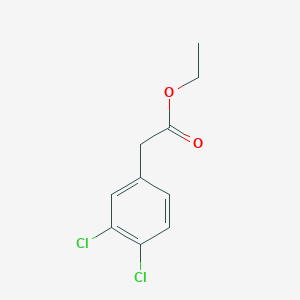
Ethyl 3,4-dichlorophenylacetate
Overview
Description
Ethyl 3,4-dichlorophenylacetate is an organic compound with the molecular formula C10H10Cl2O2. It is an ester derived from 3,4-dichlorophenylacetic acid and ethanol. This compound is known for its applications in various fields, including organic synthesis and pharmaceuticals.
Preparation Methods
Synthetic Routes and Reaction Conditions: Ethyl 3,4-dichlorophenylacetate can be synthesized through the esterification of 3,4-dichlorophenylacetic acid with ethanol in the presence of a catalyst such as sulfuric acid. The reaction typically involves heating the mixture under reflux conditions to facilitate the formation of the ester.
Industrial Production Methods: In an industrial setting, the synthesis of this compound may involve continuous flow processes to enhance efficiency and yield. The use of advanced catalysts and optimized reaction conditions can further improve the production process.
Chemical Reactions Analysis
Types of Reactions: Ethyl 3,4-dichlorophenylacetate undergoes various chemical reactions, including:
Hydrolysis: In the presence of a strong acid or base, the ester can be hydrolyzed to yield 3,4-dichlorophenylacetic acid and ethanol.
Reduction: The compound can be reduced using reducing agents such as lithium aluminum hydride to produce the corresponding alcohol.
Substitution: The chlorine atoms in the aromatic ring can undergo nucleophilic substitution reactions with suitable nucleophiles.
Common Reagents and Conditions:
Hydrolysis: Strong acids (e.g., hydrochloric acid) or bases (e.g., sodium hydroxide) under reflux conditions.
Reduction: Lithium aluminum hydride in anhydrous ether.
Substitution: Nucleophiles such as amines or thiols in the presence of a base.
Major Products:
Hydrolysis: 3,4-dichlorophenylacetic acid and ethanol.
Reduction: 3,4-dichlorophenylethanol.
Substitution: Various substituted derivatives depending on the nucleophile used.
Scientific Research Applications
Ethyl 3,4-dichlorophenylacetate has several applications in scientific research:
Chemistry: It is used as an intermediate in the synthesis of more complex organic molecules.
Biology: The compound can be used in studies involving enzyme inhibition and receptor binding.
Medicine: It serves as a precursor for the synthesis of pharmaceutical agents with potential therapeutic effects.
Industry: The compound is utilized in the production of agrochemicals and other industrial chemicals.
Mechanism of Action
The mechanism of action of ethyl 3,4-dichlorophenylacetate involves its interaction with specific molecular targets. For instance, in biological systems, it may act as an inhibitor of certain enzymes or receptors, thereby modulating biochemical pathways. The exact mechanism depends on the specific application and the molecular targets involved.
Comparison with Similar Compounds
Ethyl 3,4-dichlorophenylacetate can be compared with other similar compounds such as:
- Ethyl 2,4-dichlorophenylacetate
- Ethyl 3,5-dichlorophenylacetate
- Ethyl 3,4-dibromophenylacetate
Uniqueness: The unique positioning of the chlorine atoms on the aromatic ring of this compound imparts distinct chemical properties and reactivity compared to its analogs. This makes it particularly useful in specific synthetic and industrial applications.
Properties
IUPAC Name |
ethyl 2-(3,4-dichlorophenyl)acetate | |
---|---|---|
Source | PubChem | |
URL | https://pubchem.ncbi.nlm.nih.gov | |
Description | Data deposited in or computed by PubChem | |
InChI |
InChI=1S/C10H10Cl2O2/c1-2-14-10(13)6-7-3-4-8(11)9(12)5-7/h3-5H,2,6H2,1H3 | |
Source | PubChem | |
URL | https://pubchem.ncbi.nlm.nih.gov | |
Description | Data deposited in or computed by PubChem | |
InChI Key |
QAEBVJIVEPSFRK-UHFFFAOYSA-N | |
Source | PubChem | |
URL | https://pubchem.ncbi.nlm.nih.gov | |
Description | Data deposited in or computed by PubChem | |
Canonical SMILES |
CCOC(=O)CC1=CC(=C(C=C1)Cl)Cl | |
Source | PubChem | |
URL | https://pubchem.ncbi.nlm.nih.gov | |
Description | Data deposited in or computed by PubChem | |
Molecular Formula |
C10H10Cl2O2 | |
Source | PubChem | |
URL | https://pubchem.ncbi.nlm.nih.gov | |
Description | Data deposited in or computed by PubChem | |
DSSTOX Substance ID |
DTXSID40372051 | |
Record name | Ethyl 3,4-dichlorophenylacetate | |
Source | EPA DSSTox | |
URL | https://comptox.epa.gov/dashboard/DTXSID40372051 | |
Description | DSSTox provides a high quality public chemistry resource for supporting improved predictive toxicology. | |
Molecular Weight |
233.09 g/mol | |
Source | PubChem | |
URL | https://pubchem.ncbi.nlm.nih.gov | |
Description | Data deposited in or computed by PubChem | |
CAS No. |
6725-45-7 | |
Record name | Ethyl 3,4-dichlorophenylacetate | |
Source | EPA DSSTox | |
URL | https://comptox.epa.gov/dashboard/DTXSID40372051 | |
Description | DSSTox provides a high quality public chemistry resource for supporting improved predictive toxicology. | |
Synthesis routes and methods I
Procedure details
Synthesis routes and methods II
Procedure details
Synthesis routes and methods III
Procedure details
Retrosynthesis Analysis
AI-Powered Synthesis Planning: Our tool employs the Template_relevance Pistachio, Template_relevance Bkms_metabolic, Template_relevance Pistachio_ringbreaker, Template_relevance Reaxys, Template_relevance Reaxys_biocatalysis model, leveraging a vast database of chemical reactions to predict feasible synthetic routes.
One-Step Synthesis Focus: Specifically designed for one-step synthesis, it provides concise and direct routes for your target compounds, streamlining the synthesis process.
Accurate Predictions: Utilizing the extensive PISTACHIO, BKMS_METABOLIC, PISTACHIO_RINGBREAKER, REAXYS, REAXYS_BIOCATALYSIS database, our tool offers high-accuracy predictions, reflecting the latest in chemical research and data.
Strategy Settings
Precursor scoring | Relevance Heuristic |
---|---|
Min. plausibility | 0.01 |
Model | Template_relevance |
Template Set | Pistachio/Bkms_metabolic/Pistachio_ringbreaker/Reaxys/Reaxys_biocatalysis |
Top-N result to add to graph | 6 |
Feasible Synthetic Routes
Disclaimer and Information on In-Vitro Research Products
Please be aware that all articles and product information presented on BenchChem are intended solely for informational purposes. The products available for purchase on BenchChem are specifically designed for in-vitro studies, which are conducted outside of living organisms. In-vitro studies, derived from the Latin term "in glass," involve experiments performed in controlled laboratory settings using cells or tissues. It is important to note that these products are not categorized as medicines or drugs, and they have not received approval from the FDA for the prevention, treatment, or cure of any medical condition, ailment, or disease. We must emphasize that any form of bodily introduction of these products into humans or animals is strictly prohibited by law. It is essential to adhere to these guidelines to ensure compliance with legal and ethical standards in research and experimentation.