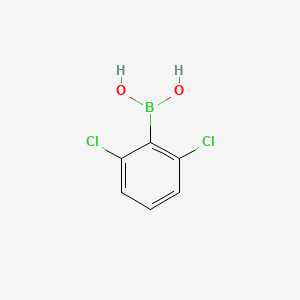
2,6-Dichlorophenylboronic Acid
Overview
Description
2,6-Dichlorophenylboronic Acid: is an organoboron compound with the molecular formula C6H5BCl2O2. It is a derivative of phenylboronic acid where two chlorine atoms are substituted at the 2 and 6 positions on the phenyl ring. This compound is commonly used in organic synthesis, particularly in Suzuki-Miyaura cross-coupling reactions, which are pivotal in forming carbon-carbon bonds.
Preparation Methods
Synthetic Routes and Reaction Conditions: 2,6-Dichlorophenylboronic Acid can be synthesized through several methods. One common method involves the reaction of 2,6-dichlorophenylmagnesium bromide with trimethyl borate, followed by hydrolysis. The reaction conditions typically include:
Reagents: 2,6-dichlorophenylmagnesium bromide, trimethyl borate
Solvent: Anhydrous ether
Temperature: Room temperature
Hydrolysis: Acidic conditions using hydrochloric acid
Another method involves the direct borylation of 2,6-dichloroiodobenzene using a palladium catalyst and bis(pinacolato)diboron under mild conditions.
Industrial Production Methods: Industrial production of this compound often employs large-scale Suzuki-Miyaura coupling reactions. The process involves:
Reagents: 2,6-dichloroiodobenzene, bis(pinacolato)diboron
Catalyst: Palladium(0) complex
Solvent: Tetrahydrofuran (THF)
Temperature: Elevated temperatures (80-100°C)
Purification: Crystallization or chromatography
Chemical Reactions Analysis
Types of Reactions: 2,6-Dichlorophenylboronic Acid undergoes various chemical reactions, including:
Suzuki-Miyaura Coupling: Forms carbon-carbon bonds with aryl halides.
Oxidation: Converts to phenols using oxidizing agents.
Substitution: Reacts with nucleophiles to replace the boronic acid group.
Common Reagents and Conditions:
Suzuki-Miyaura Coupling: Palladium catalyst, base (e.g., potassium carbonate), solvent (e.g., THF or toluene), temperature (80-100°C).
Oxidation: Hydrogen peroxide or sodium perborate in aqueous or organic solvents.
Substitution: Nucleophiles such as amines or alcohols in the presence of a base.
Major Products:
Suzuki-Miyaura Coupling: Biaryl compounds.
Oxidation: 2,6-dichlorophenol.
Substitution: Various substituted phenyl derivatives.
Scientific Research Applications
2,6-Dichlorophenylboronic Acid has numerous applications in scientific research:
Chemistry: Used as a reagent in Suzuki-Miyaura coupling to synthesize biaryl compounds, which are important in pharmaceuticals and agrochemicals.
Biology: Employed in the synthesis of biologically active molecules, including enzyme inhibitors and receptor modulators.
Medicine: Utilized in the development of drug candidates, particularly those targeting cancer and inflammatory diseases.
Industry: Applied in the production of advanced materials, such as polymers and electronic components.
Mechanism of Action
The primary mechanism of action for 2,6-Dichlorophenylboronic Acid in Suzuki-Miyaura coupling involves:
Oxidative Addition: The palladium catalyst inserts into the carbon-halogen bond of the aryl halide.
Transmetalation: The aryl group from the boronic acid is transferred to the palladium complex.
Reductive Elimination: The palladium complex forms the new carbon-carbon bond and regenerates the palladium(0) catalyst.
Comparison with Similar Compounds
- 2,4-Dichlorophenylboronic Acid
- 2,5-Dichlorophenylboronic Acid
- 2,6-Dimethylphenylboronic Acid
Comparison:
- 2,6-Dichlorophenylboronic Acid is unique due to the specific positioning of chlorine atoms, which influences its reactivity and selectivity in coupling reactions.
- 2,4-Dichlorophenylboronic Acid and 2,5-Dichlorophenylboronic Acid have different substitution patterns, affecting their steric and electronic properties.
- 2,6-Dimethylphenylboronic Acid has methyl groups instead of chlorine, leading to different reactivity and applications.
Properties
IUPAC Name |
(2,6-dichlorophenyl)boronic acid | |
---|---|---|
Source | PubChem | |
URL | https://pubchem.ncbi.nlm.nih.gov | |
Description | Data deposited in or computed by PubChem | |
InChI |
InChI=1S/C6H5BCl2O2/c8-4-2-1-3-5(9)6(4)7(10)11/h1-3,10-11H | |
Source | PubChem | |
URL | https://pubchem.ncbi.nlm.nih.gov | |
Description | Data deposited in or computed by PubChem | |
InChI Key |
CXDPUSMFYPQXCV-UHFFFAOYSA-N | |
Source | PubChem | |
URL | https://pubchem.ncbi.nlm.nih.gov | |
Description | Data deposited in or computed by PubChem | |
Canonical SMILES |
B(C1=C(C=CC=C1Cl)Cl)(O)O | |
Source | PubChem | |
URL | https://pubchem.ncbi.nlm.nih.gov | |
Description | Data deposited in or computed by PubChem | |
Molecular Formula |
C6H5BCl2O2 | |
Source | PubChem | |
URL | https://pubchem.ncbi.nlm.nih.gov | |
Description | Data deposited in or computed by PubChem | |
DSSTOX Substance ID |
DTXSID30370223 | |
Record name | 2,6-Dichlorophenylboronic Acid | |
Source | EPA DSSTox | |
URL | https://comptox.epa.gov/dashboard/DTXSID30370223 | |
Description | DSSTox provides a high quality public chemistry resource for supporting improved predictive toxicology. | |
Molecular Weight |
190.82 g/mol | |
Source | PubChem | |
URL | https://pubchem.ncbi.nlm.nih.gov | |
Description | Data deposited in or computed by PubChem | |
CAS No. |
73852-17-2 | |
Record name | 2,6-Dichlorophenylboronic Acid | |
Source | EPA DSSTox | |
URL | https://comptox.epa.gov/dashboard/DTXSID30370223 | |
Description | DSSTox provides a high quality public chemistry resource for supporting improved predictive toxicology. | |
Record name | 2,6-Dichlorobenzeneboronic acid | |
Source | European Chemicals Agency (ECHA) | |
URL | https://echa.europa.eu/information-on-chemicals | |
Description | The European Chemicals Agency (ECHA) is an agency of the European Union which is the driving force among regulatory authorities in implementing the EU's groundbreaking chemicals legislation for the benefit of human health and the environment as well as for innovation and competitiveness. | |
Explanation | Use of the information, documents and data from the ECHA website is subject to the terms and conditions of this Legal Notice, and subject to other binding limitations provided for under applicable law, the information, documents and data made available on the ECHA website may be reproduced, distributed and/or used, totally or in part, for non-commercial purposes provided that ECHA is acknowledged as the source: "Source: European Chemicals Agency, http://echa.europa.eu/". Such acknowledgement must be included in each copy of the material. ECHA permits and encourages organisations and individuals to create links to the ECHA website under the following cumulative conditions: Links can only be made to webpages that provide a link to the Legal Notice page. | |
Synthesis routes and methods
Procedure details
Retrosynthesis Analysis
AI-Powered Synthesis Planning: Our tool employs the Template_relevance Pistachio, Template_relevance Bkms_metabolic, Template_relevance Pistachio_ringbreaker, Template_relevance Reaxys, Template_relevance Reaxys_biocatalysis model, leveraging a vast database of chemical reactions to predict feasible synthetic routes.
One-Step Synthesis Focus: Specifically designed for one-step synthesis, it provides concise and direct routes for your target compounds, streamlining the synthesis process.
Accurate Predictions: Utilizing the extensive PISTACHIO, BKMS_METABOLIC, PISTACHIO_RINGBREAKER, REAXYS, REAXYS_BIOCATALYSIS database, our tool offers high-accuracy predictions, reflecting the latest in chemical research and data.
Strategy Settings
Precursor scoring | Relevance Heuristic |
---|---|
Min. plausibility | 0.01 |
Model | Template_relevance |
Template Set | Pistachio/Bkms_metabolic/Pistachio_ringbreaker/Reaxys/Reaxys_biocatalysis |
Top-N result to add to graph | 6 |
Feasible Synthetic Routes
Disclaimer and Information on In-Vitro Research Products
Please be aware that all articles and product information presented on BenchChem are intended solely for informational purposes. The products available for purchase on BenchChem are specifically designed for in-vitro studies, which are conducted outside of living organisms. In-vitro studies, derived from the Latin term "in glass," involve experiments performed in controlled laboratory settings using cells or tissues. It is important to note that these products are not categorized as medicines or drugs, and they have not received approval from the FDA for the prevention, treatment, or cure of any medical condition, ailment, or disease. We must emphasize that any form of bodily introduction of these products into humans or animals is strictly prohibited by law. It is essential to adhere to these guidelines to ensure compliance with legal and ethical standards in research and experimentation.