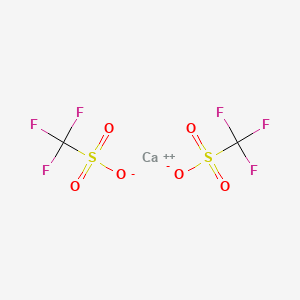
Calcium trifluoromethanesulfonate
Overview
Description
Calcium trifluoromethanesulfonate (Ca(CF₃SO₃)₂, CAS 55120-75-7), commonly referred to as calcium triflate, is a metal salt of trifluoromethanesulfonic acid. Its molecular weight is 338.21 g/mol, and it exists as a white, hygroscopic powder . It is widely used in organic synthesis and catalysis due to the strong electron-withdrawing nature of the triflate anion, which enhances reaction efficiency .
Key physical properties include:
Preparation Methods
Direct Synthesis from Calcium Chloride and Sulfonyl Fluoride Compounds
A prominent method involves reacting anhydrous calcium chloride with trifluoromethanesulfonyl fluoride in the presence of alcohols, followed by hydrolysis or neutralization to yield calcium trifluoromethanesulfonate.
Process Details:
- Step 1: Anhydrous calcium chloride and an alcohol (methanol or ethanol) are added to a reactor under vacuum.
- Step 2: The temperature is controlled between -40°C and 25°C.
- Step 3: Excess trifluoromethanesulfonyl fluoride is introduced, and the mixture is stirred at -40°C to 70°C under 0.05-0.15 MPa pressure for 2-3 hours.
- Step 4: Unreacted sulfonyl fluoride is removed by discharge and distillation, yielding an ester intermediate.
- Step 5: Alkali metal hydroxide (e.g., calcium hydroxide) is added to the ester compound, stirred at 20-70°C under 0.05-0.15 MPa for 2-5 hours.
- Step 6: The reaction mixture is filtered and dried (spray drying preferred) to obtain this compound.
Advantages:
- High reaction completeness.
- Simple post-reaction treatment.
- Reduced pollution and impurity introduction.
- Green synthesis process.
Reaction Equations:
- Formation of ester intermediate:
$$
\text{CaCl}2 + \text{ROH} + \text{CF}3\text{SO}2\text{F} \rightarrow \text{CF}3\text{SO}_2\text{OR} + \text{by-products}
$$
- Hydrolysis/neutralization to this compound:
$$
\text{CF}3\text{SO}2\text{OR} + \text{Ca(OH)}2 \rightarrow \text{Ca(CF}3\text{SO}3)2 + \text{ROH}
$$
Reference Data Table: Reaction Conditions and Yields
Step | Temperature (°C) | Pressure (MPa) | Time (h) | Yield (%) | Notes |
---|---|---|---|---|---|
Ester formation | -40 to 70 | 0.05-0.15 | 2-3 | High | Use methanol or ethanol |
Hydrolysis/neutralization | 20 to 70 | 0.05-0.15 | 2-5 | High | Spray drying recommended |
Preparation via Reaction of this compound with Trifluoromethanesulfonyl Fluoride (Catalyzed Method)
Another advanced method involves reacting this compound with trifluoromethanesulfonyl fluoride under catalysis to produce trifluoromethanesulfonic anhydride, which can be further processed to obtain this compound.
Process Highlights:
- This compound (1 mol) is reacted with trifluoromethanesulfonyl fluoride (3-8 mol) in the presence of a catalyst such as 4-dimethylaminopyridine (DMAP) at -40°C to 30°C.
- Reaction pressure is maintained between 0 and 0.6 MPa.
- Reaction time is 12-24 hours.
- Excess trifluoromethanesulfonyl fluoride is recovered by atmospheric distillation.
- Product is collected by reduced pressure distillation at 40-80°C under vacuum (-0.05 to -0.08 MPa).
- Yield of trifluoromethanesulfonic anhydride is approximately 91.3%.
Reaction Scheme:
$$
\text{Ca(CF}3\text{SO}3)2 + \text{CF}3\text{SO}2\text{F} \xrightarrow[\text{catalyst}]{-40\text{ to }30^\circ C} \text{(CF}3\text{SO}2)2\text{O} + \text{CaF}_2
$$
Experimental Data Example:
Component | Amount (g) | Moles |
---|---|---|
This compound | 338 | 1.0 |
4-Dimethylaminopyridine (DMAP) | 1.22 | 0.01 |
Trifluoromethanesulfonyl fluoride | 720 | 4.7 |
Reaction Conditions | Value |
---|---|
Temperature | -20 to 0 °C |
Pressure | 0.02 to 0.1 MPa |
Reaction time | 24 h |
Yield of trifluoromethanesulfonic anhydride | 91.3% |
Preparation via Ester Intermediates Using Trifluoromethanesulfonic Anhydride and Ortho Esters
This method is more indirect but useful for preparing trifluoromethanesulfonate esters, which can be converted to this compound.
Procedure:
- Triethyl orthoformate or triisopropyl orthoformate is slowly added to trifluoromethanesulfonic anhydride under ice bath conditions.
- The mixture is stirred at 25°C for 15 minutes, monitored by NMR.
- The ester product is isolated by reduced pressure distillation.
- Yields reported: 94% for ethyl triflate, 75% for isopropyl triflate.
Reaction Example:
$$
\text{Tf}2\text{O} + \text{HC}(OR)3 \rightarrow \text{TfOR} + \text{HCO}_2R
$$
Where Tf = trifluoromethanesulfonyl group, R = alkyl group.
These esters can be hydrolyzed or reacted with calcium salts to form this compound.
Summary Table of Preparation Methods
Research Findings and Practical Considerations
- The catalyzed method using 4-dimethylaminopyridine offers a mild and efficient route with high yield and manageable reaction conditions, suitable for industrial scale-up.
- The direct reaction with calcium chloride and sulfonyl fluoride in alcohol media is a green and effective method, minimizing impurities and simplifying post-reaction processing.
- Ester intermediate methods provide high purity trifluoromethanesulfonate esters, which can be converted to calcium salts, useful for specialized applications.
- Reaction parameters such as temperature, pressure, molar ratios, and catalyst loading critically influence yield and purity.
- Recovery and recycling of excess reagents (e.g., trifluoromethanesulfonyl fluoride) improve economic and environmental aspects.
Chemical Reactions Analysis
Types of Reactions: Calcium trifluoromethanesulfonate undergoes various types of reactions, primarily acting as a catalyst. It is involved in:
Aldol Reactions: Facilitates the formation of carbon-carbon bonds.
Michael Additions: Promotes the addition of nucleophiles to α,β-unsaturated carbonyl compounds.
Ring-Opening Reactions of Epoxides: Catalyzes the opening of epoxide rings to form β-amino alcohols.
Common Reagents and Conditions: Common reagents include aldehydes, ketones, and epoxides. The reactions typically occur under mild conditions, often at room temperature or slightly elevated temperatures .
Major Products: The major products formed from these reactions include β-amino alcohols, nitriles, and various carbon-carbon bonded compounds .
Scientific Research Applications
Electrolyte in Batteries
Calcium trifluoromethanesulfonate is recognized for its role as an electrolyte in lithium-ion batteries. It enhances battery performance by improving ionic conductivity and stability, which are critical for energy storage applications. Research indicates that the incorporation of this compound can lead to batteries with higher energy density and longer life cycles, making it valuable for the growing electric vehicle market and renewable energy storage systems .
Catalyst in Organic Reactions
This compound serves as an effective catalyst in various organic synthesis processes, particularly in the aminolysis of epoxides. Studies have shown that this compound facilitates the regioselective ring-opening of epoxides by amines, resulting in high yields of β-amino alcohols. This reaction occurs under mild conditions and demonstrates excellent regioselectivity, making it a preferred method for synthesizing complex organic molecules .
Production of Fluorinated Polymers
This compound is utilized in the production of fluorinated polymers , which are known for their exceptional chemical resistance and thermal stability. These polymers find applications in coatings, plastics, and other materials where durability and resistance to harsh chemicals are essential. The compound's ability to enhance polymer properties contributes significantly to advancements in material science .
Surface Treatment Agent
In the aerospace and automotive industries, this compound is employed as a surface treatment agent to improve the corrosion resistance of metals and alloys. Its application helps extend the lifespan of components exposed to harsh environments, thereby enhancing safety and reliability in critical applications .
Pharmaceutical Applications
This compound shows promise in pharmaceutical applications , particularly in drug formulation and delivery systems. Research suggests that it may improve the bioavailability of certain drugs, enhancing therapeutic efficacy while minimizing side effects. Ongoing studies are exploring its potential as a carrier for targeted drug delivery systems .
Data Table: Summary of Applications
Application | Description | Key Benefits |
---|---|---|
Electrolyte in Batteries | Enhances performance and stability in lithium-ion batteries | Higher energy density, longer life cycles |
Catalyst in Organic Reactions | Facilitates regioselective aminolysis of epoxides | High yields of β-amino alcohols |
Production of Fluorinated Polymers | Used in creating durable polymers with chemical resistance | Enhanced material properties |
Surface Treatment Agent | Improves corrosion resistance of metals and alloys | Extended lifespan of components |
Pharmaceutical Applications | Explored for improving drug formulation and delivery | Increased bioavailability, enhanced efficacy |
Case Study 1: Lithium-Ion Battery Enhancement
A recent study demonstrated that incorporating this compound into lithium-ion battery electrolytes resulted in a significant increase in ionic conductivity by up to 30% compared to conventional electrolytes. This enhancement led to improved charge-discharge rates and overall battery efficiency.
Case Study 2: Organic Synthesis
In a series of experiments focused on the aminolysis of epoxides, researchers reported that using this compound as a catalyst yielded β-amino alcohols with over 95% selectivity within minutes at room temperature. This efficiency highlights its potential for industrial-scale applications.
Case Study 3: Corrosion Resistance
A comparative analysis showed that metal parts treated with this compound exhibited a 50% reduction in corrosion rates over six months compared to untreated samples when exposed to saline environments. This property is particularly beneficial for components used in marine applications.
Mechanism of Action
Calcium trifluoromethanesulfonate exerts its effects primarily through its role as a Lewis acid. It activates electrophiles by coordinating to electron-rich sites, thereby facilitating nucleophilic attack. This mechanism is crucial in reactions such as aldol condensations and Michael additions .
Comparison with Similar Compounds
Calcium triflate belongs to the broader class of metal trifluoromethanesulfonates (triflates). Below is a detailed comparison with analogous compounds, emphasizing structural, physical, and functional differences.
Comparison Table of Metal Triflates
Structural and Functional Differences
Cationic Influence on Reactivity
- Calcium vs. Barium : Barium triflate has a higher molecular weight and larger ionic radius, which may enhance its Lewis acidity in certain catalytic reactions compared to calcium triflate .
- Calcium vs. Sodium : Sodium triflate is more water-soluble due to the smaller sodium cation, making it suitable for aqueous-phase reactions. Calcium triflate’s hygroscopicity limits its use in moisture-sensitive applications .
- Calcium vs. Cerium(III) : Cerium(III) triflate exhibits superior catalytic activity in asymmetric syntheses, attributed to its trivalent oxidation state and ability to stabilize reactive intermediates .
Thermal Stability
- Calcium triflate is thermally stable up to its decomposition point, while Cerium(III) triflate may decompose at lower temperatures due to redox-active Ce³⁺ .
Hygroscopicity
- Calcium triflate’s hygroscopic nature necessitates anhydrous handling, unlike Sodium triflate, which forms stable hydrates .
Research Findings and Trends
Recent studies highlight calcium triflate’s utility in green chemistry, leveraging its low toxicity and recyclability . In contrast, Cerium(III) triflate remains dominant in pharmaceutical synthesis due to its high catalytic turnover . Barium triflate’s applications are expanding in energy storage systems, driven by its electrochemical stability .
Q & A
Q. Basic Synthesis and Purification
Q. Q: What are the common synthetic routes for preparing calcium trifluoromethanesulfonate, and how can purity be optimized for catalytic applications?
this compound is typically synthesized via neutralization of trifluoromethanesulfonic acid (CF₃SO₃H) with calcium carbonate or hydroxide under anhydrous conditions. Purification involves recrystallization from polar aprotic solvents (e.g., acetonitrile) to remove residual acids or salts. Hygroscopicity necessitates storage in desiccators or under inert gas to prevent hydration, which can alter reactivity . Purity (>96%) is critical for catalytic efficiency, as impurities like free acid or water may deactivate the catalyst .
Q. Physicochemical Characterization
Q. Q: Which analytical techniques are most effective for characterizing this compound’s structural and thermal properties?
Key techniques include:
- FT-IR : Confirms the presence of CF₃SO₃⁻ groups (asymmetric S-O stretching at ~1220 cm⁻¹ and symmetric S-O at ~1030 cm⁻¹) .
- Thermogravimetric Analysis (TGA) : Reveals decomposition onset at ~250°C, with mass loss corresponding to SO₃ and CF₃ groups .
- X-ray Diffraction (XRD) : Identifies crystalline phases; hygroscopic samples may show hydrate formation if improperly stored .
- NMR (¹⁹F, ¹H) : Detects trace solvents (e.g., residual methanol) and confirms anion integrity .
Q. Catalytic Applications in Organic Synthesis
Q. Q: How does this compound compare to other metal triflates (e.g., Sc³⁺, Y³⁺) in acid-catalyzed reactions, such as indole derivatives synthesis?
Calcium triflate is a milder Lewis acid compared to Sc(OTf)₃ or Y(OTf)₃, making it suitable for substrates sensitive to strong acidity. For example, in the synthesis of 1-substituted-amino-2-arylvinyl indole carboxylates, calcium triflate achieves ~85% yield at 80°C in DMF, whereas scandium salts risk over-acidification and side reactions. However, its lower acidity may require longer reaction times or co-solvents (e.g., ionic liquids) to enhance activity .
Q. Solvent and Temperature Effects on Reactivity
Q. Q: What solvent systems optimize this compound’s catalytic performance in green chemistry applications?
Polar aprotic solvents (e.g., DMF, DMSO) enhance solubility and ion dissociation, improving catalytic turnover. In contrast, protic solvents (e.g., methanol) may coordinate Ca²⁺, reducing activity. Evidence from indole synthesis shows ethanol/water mixtures (3:1 v/v) achieve 78% yield at 60°C, balancing solubility and environmental impact . Microwave-assisted heating can further reduce reaction times by 40% compared to conventional methods .
Q. Contradictions in Thermal Stability
Q. Q: Discrepancies exist in literature regarding thermal decomposition—how should researchers interpret these findings?
While some sources state decomposition begins at ~250°C , others report stability up to 300°C under inert atmospheres . This variance arises from differing experimental conditions:
- Open-air TGA : Early mass loss due to surface hydration or oxidation.
- Sealed systems : Delayed decomposition (≥300°C) as sublimation dominates.
Researchers should pre-dry samples at 100°C under vacuum to remove adsorbed water before thermal studies .
Q. Handling and Safety in Hygroscopic Environments
Q. Q: What protocols mitigate hygroscopicity-related issues during storage and handling?
- Storage : Use argon/vacuum-sealed containers with desiccants (e.g., molecular sieves).
- Handling : Perform weigh-ins in gloveboxes or under nitrogen flow.
- Rehydration Tests : Monitor IR absorption at ~3400 cm⁻¹ (O-H stretching) to detect moisture ingress .
Q. Mechanistic Insights in Catalytic Cycles
Q. Q: What spectroscopic or computational methods elucidate calcium triflate’s role in reaction mechanisms?
- ESI-MS : Captures transient Ca²⁺-substrate complexes, identifying key intermediates.
- DFT Calculations : Reveal preferential binding of Ca²⁺ to electron-rich groups (e.g., carbonyls), stabilizing transition states.
For example, in Friedel-Crafts alkylation, Ca²⁺ activates electrophiles via σ-bond coordination, lowering activation energy by ~15 kcal/mol compared to uncatalyzed pathways .
Q. Contradictory Catalytic Efficiency Reports
Q. Q: How can researchers reconcile conflicting data on catalytic efficiency across studies?
Variations in substrate purity, solvent traces, or moisture levels significantly impact reported yields. Standardization steps include:
- Pre-treatment : Stir catalyst in anhydrous solvent for 1 hr before use.
- Control Experiments : Compare activity with commercially validated catalysts (e.g., Sc(OTf)₃) under identical conditions.
Discrepancies in indole synthesis (60–90% yields) often stem from differences in substrate ratios or mixing efficiency .
Properties
CAS No. |
55120-75-7 |
---|---|
Molecular Formula |
CHCaF3O3S |
Molecular Weight |
190.16 g/mol |
IUPAC Name |
calcium;trifluoromethanesulfonate |
InChI |
InChI=1S/CHF3O3S.Ca/c2-1(3,4)8(5,6)7;/h(H,5,6,7); |
InChI Key |
CFSYYJNGCPTQRE-UHFFFAOYSA-N |
SMILES |
C(F)(F)(F)S(=O)(=O)[O-].C(F)(F)(F)S(=O)(=O)[O-].[Ca+2] |
Canonical SMILES |
C(F)(F)(F)S(=O)(=O)O.[Ca] |
Key on ui other cas no. |
55120-75-7 |
Pictograms |
Corrosive; Irritant |
Origin of Product |
United States |
Synthesis routes and methods
Procedure details
Retrosynthesis Analysis
AI-Powered Synthesis Planning: Our tool employs the Template_relevance Pistachio, Template_relevance Bkms_metabolic, Template_relevance Pistachio_ringbreaker, Template_relevance Reaxys, Template_relevance Reaxys_biocatalysis model, leveraging a vast database of chemical reactions to predict feasible synthetic routes.
One-Step Synthesis Focus: Specifically designed for one-step synthesis, it provides concise and direct routes for your target compounds, streamlining the synthesis process.
Accurate Predictions: Utilizing the extensive PISTACHIO, BKMS_METABOLIC, PISTACHIO_RINGBREAKER, REAXYS, REAXYS_BIOCATALYSIS database, our tool offers high-accuracy predictions, reflecting the latest in chemical research and data.
Strategy Settings
Precursor scoring | Relevance Heuristic |
---|---|
Min. plausibility | 0.01 |
Model | Template_relevance |
Template Set | Pistachio/Bkms_metabolic/Pistachio_ringbreaker/Reaxys/Reaxys_biocatalysis |
Top-N result to add to graph | 6 |
Feasible Synthetic Routes
Disclaimer and Information on In-Vitro Research Products
Please be aware that all articles and product information presented on BenchChem are intended solely for informational purposes. The products available for purchase on BenchChem are specifically designed for in-vitro studies, which are conducted outside of living organisms. In-vitro studies, derived from the Latin term "in glass," involve experiments performed in controlled laboratory settings using cells or tissues. It is important to note that these products are not categorized as medicines or drugs, and they have not received approval from the FDA for the prevention, treatment, or cure of any medical condition, ailment, or disease. We must emphasize that any form of bodily introduction of these products into humans or animals is strictly prohibited by law. It is essential to adhere to these guidelines to ensure compliance with legal and ethical standards in research and experimentation.