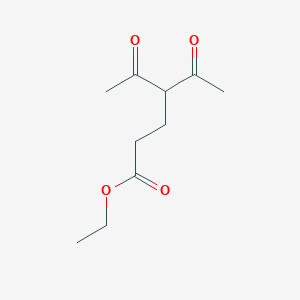
Ethyl 4-acetyl-5-oxohexanoate
Overview
Description
Ethyl 4-acetyl-5-oxohexanoate (CAS: 2832-10-2) is a β-dicarbonyl compound with the molecular formula C₁₀H₁₆O₄ and a molecular weight of 200.23 g/mol . Its IUPAC name reflects its structure: an ethyl ester at the terminal carboxyl group, a 4-acetyl substituent, and a 5-oxo (keto) group. Key synonyms include 4-Acetyl-5-oxohexanoic acid ethyl ester and Ethyl 4,4-diacetylbutyrate .
Preparation Methods
Preparation Methods of Ethyl 4-acetyl-5-oxohexanoate
Michael Addition Reaction Using Acetone and Ethyl Acrylate
The most prominent and industrially relevant method for preparing this compound involves a Michael addition reaction between acetone and ethyl acrylate catalyzed by bifunctional catalysts possessing both acidic and basic centers.
- Acetone is dissolved in a suitable solvent and heated to a controlled temperature (20–120 °C).
- A catalyst such as potassium hydrogen phosphate (K2HPO4), sodium bicarbonate (NaHCO3), ethylamine hydrochloride, or diethylamine hydrochloride is added.
- Ethyl acrylate solution is added dropwise over several hours (typically 3 hours) under reflux conditions.
- After completion, the reaction mixture is worked up by removing residual acetone, solvent, and unreacted acrylate via evaporation under reduced pressure.
- The product, this compound, is isolated with moderate yield and high selectivity.
Catalyst | Temperature (°C) | Reaction Time (h) | Yield (%) | Selectivity (%) | Notes |
---|---|---|---|---|---|
K2HPO4 | 60 | 3 | 34 | 90 | Optimal temperature for selectivity |
Ethylamine hydrochloride | 60 | 3 | 30 | 90 | Comparable yield and selectivity |
K2HPO4 | 80 | 3 | 29 | 58 | Higher temp reduces selectivity due to side reactions |
- The Michael addition selectivity exceeds 90% under optimized conditions, with yields around 30-37% reported.
- Higher temperatures (>60 °C) increase catalyst activity but promote side reactions, lowering selectivity.
- Mild reaction conditions reduce energy consumption.
- Use of inexpensive raw materials (acetone and ethyl acrylate).
- Avoidance of expensive or unstable catalysts.
- Small, economical equipment suitable for scale-up.
- Moderate yields require optimization for industrial scale.
- Side reactions at elevated temperatures can reduce product purity.
Solvent-Free Catalytic Michael Addition Using 1,3-Cyclohexanedione and Ethanol
An alternative method reported involves the reaction of 1,3-cyclohexanedione with ethanol in the presence of onium tetrafluoroborate catalysts under solvent-free conditions at 100 °C for 16 hours.
- The reaction proceeds via Michael addition to form ethyl 5-oxohexanoate derivatives.
- The product is isolated by column chromatography with a yield of 76%.
- Although this method achieves higher yield, the catalyst (onium tetrafluoroborate) is expensive and prone to deactivation, limiting industrial applicability.
- The long reaction time and high temperature also pose challenges for scale-up.
Related Ester Derivatives Preparation (Supporting Methods)
Research on related compounds such as 2-methoxythis compound provides insight into similar Michael addition strategies using acrylate esters and diketones with potassium carbonate catalysis.
- For example, 2-methoxythis compound was synthesized by reacting ethylene glycol methyl ether acrylate with 2,4-pentanedione in the presence of potassium carbonate, yielding 58-76% after vacuum distillation.
- These methods highlight the versatility of Michael addition for preparing various ester analogues of 4-acetyl-5-oxohexanoate.
Comparative Analysis of Preparation Methods
Method | Catalyst Type | Reaction Conditions | Yield (%) | Selectivity (%) | Industrial Viability |
---|---|---|---|---|---|
Michael addition (acetone + ethyl acrylate) | K2HPO4, NaHCO3, amine salts | 20-60 °C, 3-8 h, solvent or solvent-free | 30-37 | >90 | High (cost-effective, mild) |
Michael addition (1,3-cyclohexanedione + ethanol) | Onium tetrafluoroborate | 100 °C, 16 h, solvent-free | 76 | Not specified | Low (expensive catalyst) |
Michael addition (acrylate esters + diketones) | Potassium carbonate | Mild, vacuum distillation | 58-76 | Not specified | Moderate (research scale) |
Summary of Research Findings
- The Michael addition of acetone to ethyl acrylate catalyzed by bifunctional catalysts such as K2HPO4 is the most practical and scalable method for preparing this compound, balancing yield, selectivity, and cost.
- Catalyst choice and reaction temperature critically influence selectivity and yield; mild temperatures (~60 °C) favor high selectivity and moderate yields.
- Alternative methods using expensive catalysts or longer reaction times offer higher yields but are less industrially feasible.
- Related ester derivatives can be synthesized by similar Michael addition strategies, expanding the scope of this chemistry.
Chemical Reactions Analysis
Types of Reactions
Ethyl 4-acetyl-5-oxohexanoate undergoes various chemical reactions, including:
Oxidation: This compound can be oxidized to form corresponding carboxylic acids or ketones.
Reduction: Reduction reactions can convert the keto group to a hydroxyl group, forming alcohols.
Substitution: Nucleophilic substitution reactions can replace the ethoxy group with other functional groups.
Common Reagents and Conditions
Oxidation: Common oxidizing agents include potassium permanganate (KMnO4) and chromium trioxide (CrO3).
Reduction: Reducing agents such as sodium borohydride (NaBH4) and lithium aluminum hydride (LiAlH4) are used.
Substitution: Nucleophiles like amines or thiols can be used in substitution reactions.
Major Products Formed
Oxidation: Carboxylic acids or ketones.
Reduction: Alcohols.
Substitution: Various substituted derivatives depending on the nucleophile used.
Scientific Research Applications
Pharmaceutical Applications
Ethyl 4-acetyl-5-oxohexanoate is primarily recognized for its role as an intermediate in the synthesis of various pharmaceutical compounds. Its structural characteristics allow it to participate in several chemical reactions, making it valuable in drug development.
- Synthesis of Antitumor Agents : This compound has been used to synthesize curcumin analogs that exhibit potential antiandrogenic activity. These analogs are being explored for their efficacy against prostate cancer, as they can act as androgen receptor antagonists .
- Biocatalysis : In the pharmaceutical industry, this compound serves as a substrate for biocatalytic processes. Biocatalysis has been recognized for its efficiency in producing chiral intermediates which are crucial in the synthesis of active pharmaceutical ingredients (APIs) .
- Drug Metabolite Studies : The compound is also utilized in studies focusing on the biosynthesis of drug metabolites, aiding researchers in understanding drug interactions and metabolism .
Organic Synthesis
This compound is employed in organic synthesis due to its functional groups that facilitate various chemical transformations.
- Aldol Reactions : It can participate in aldol reactions to form β-hydroxy ketones, which are valuable intermediates in organic chemistry .
- Formation of Heterocycles : The compound can be used to synthesize heterocyclic compounds through cyclization reactions, expanding its utility in creating complex molecular architectures .
Case Study 1: Synthesis of Curcumin Analogues
Research has demonstrated that this compound can be modified to produce curcumin analogs with enhanced biological activity. A study published in the Journal of Medicinal Chemistry highlighted the synthesis and screening of these analogs against prostate cancer cell lines, showing promising results that warrant further investigation into their therapeutic potential .
Case Study 2: Biocatalytic Applications
A comprehensive study on biocatalysis highlighted the use of this compound as a substrate for enzyme-catalyzed reactions. The study indicated that using this compound could significantly improve yields and selectivity in the production of chiral compounds necessary for pharmaceuticals .
Mechanism of Action
The mechanism of action of ethyl 4-acetyl-5-oxohexanoate involves its interaction with specific molecular targets and pathways. For instance, in the synthesis of curcumin analogs, the compound acts as a key intermediate, facilitating the formation of the final product through a series of chemical reactions. The molecular targets and pathways involved depend on the specific application and the nature of the reactions it undergoes .
Comparison with Similar Compounds
Ethyl 4-acetyl-5-oxohexanoate shares functional groups with several esters and β-dicarbonyl compounds. Below is a detailed comparison:
Ethyl 5-Oxohexanoate (CAS: 13984-57-1)
- Formula : C₈H₁₄O₃
- Molecular Weight : 158.19 g/mol .
- Key Differences: Lacks the 4-acetyl group, reducing steric hindrance and reactivity in enolate formation.
- Applications : Used as a simpler β-keto ester in nucleophilic acyl substitutions .
Mthis compound (CAS: 13984-53-7)
- Formula : C₉H₁₄O₄
- Molecular Weight : 186.20 g/mol .
- Key Differences : The methyl ester group increases polarity compared to the ethyl variant, altering solubility and hydrolysis rates.
- Reactivity : Demonstrates similar β-dicarbonyl reactivity but may exhibit slower ester hydrolysis in basic conditions due to reduced steric protection .
4-Acetyl-5-Oxohexanoic Acid (CAS: 54605-46-8)
- Formula : C₈H₁₂O₄
- Molecular Weight : 172.18 g/mol .
- Key Differences : The free carboxylic acid enhances water solubility and acidity (pKa ~4.5–5.0), making it suitable for pH-dependent reactions.
- Applications : Used in metal chelation studies due to its deprotonated carboxylate .
Ethyl 3-Oxohexanoate (CAS: 7152-15-0)
- Formula : C₈H₁₄O₃
- Molecular Weight : 158.19 g/mol .
- Key Differences: The keto group at position 3 instead of 5 reduces conjugation with the ester, limiting enolization and tautomerism .
Comparative Data Table
Reactivity and Stability Insights
- Enolization: this compound’s dual carbonyl groups promote keto-enol tautomerism, enhancing its utility in cyclocondensation reactions (e.g., pyrrole formation) .
- Steric Effects: The 4-acetyl group introduces steric hindrance, slowing nucleophilic attacks compared to Ethyl 5-oxohexanoate .
- Solvent-Free Reactions : Demonstrates high reactivity in silica gel-mediated oxidative C–O coupling due to β-dicarbonyl activation .
Biological Activity
Ethyl 4-acetyl-5-oxohexanoate, a compound with a significant presence in synthetic organic chemistry, has garnered attention for its potential biological activities, particularly in the context of cancer research. This article explores the biological activity of this compound, focusing on its cytotoxic effects, antiandrogenic properties, and its role as a precursor in the synthesis of curcumin analogs.
This compound can be characterized by its molecular formula and is classified as an ester and ketone. Its structure includes two acetyl groups and a five-membered carbon chain, which is essential for its biological activity.
Cytotoxicity
Research indicates that this compound exhibits notable cytotoxic effects against various cancer cell lines. In particular, it has been evaluated for its activity against prostate cancer cell lines LNCaP and PC-3. The following table summarizes the IC50 values from studies assessing its cytotoxicity:
Compound | Cell Line | IC50 (μM) |
---|---|---|
This compound | LNCaP | 1.3 |
This compound | PC-3 | 1.1 |
These values suggest that the compound has a strong inhibitory effect on cell growth, with IC50 values below the critical threshold of 4 μM, indicating significant biological activity .
Antiandrogenic Activity
In addition to its cytotoxic properties, this compound has been implicated in antiandrogenic activity. A study focused on curcumin analogs revealed that compounds derived from this compound demonstrated antiandrogenic effects in LNCaP cells. The structural activity relationship (SAR) established that specific substitutions on the phenyl rings enhance this activity:
- Unsubstituted C-2' positions on phenyl rings are favorable.
- Substitutions at C-3' and C-4' positions with methoxy or hydroxy groups significantly enhance antiandrogenic activity.
- Linker length impacts both cytotoxicity and antiandrogenic activity; elongation tends to decrease effectiveness .
Study on Curcumin Analogs
A pivotal study synthesized various curcumin analogs using this compound as a starting material. The study found that certain analogs exhibited enhanced cytotoxicity against prostate cancer cells compared to traditional curcumin, highlighting the potential of modifying linkers and substituents to optimize biological activity .
The mechanisms through which this compound exerts its effects are still under investigation. However, it is believed that the compound may interfere with androgen receptor signaling pathways, thus inhibiting cancer cell proliferation .
Q & A
Basic Research Questions
Q. What are the established synthetic routes for Ethyl 4-acetyl-5-oxohexanoate, and how do reaction conditions influence yield and purity?
this compound is commonly synthesized via condensation reactions. For example, methyl acrylate and acetylacetone can be condensed using sodium ethoxide as a base, yielding ~55–62% product, though contamination with ethyl esters may occur . Alternative methods include BF3·(OEt)2-catalyzed cyclization in CH2Cl2, followed by purification via preparative C18 reversed-phase LC chromatography (yield: ~50.7%) . Key variables include stoichiometry, catalyst choice, and reaction time. Researchers should monitor reactions using UPLC and confirm purity via <sup>1</sup>H/<sup>13</sup>C NMR .
Q. How can researchers optimize purification strategies for this compound to minimize byproducts?
Post-synthesis purification often involves fractional distillation (e.g., spinning-band columns for high-boiling-point compounds) or chromatographic methods. Preparative LC with water/acetonitrile gradients effectively isolates the compound from unreacted diketones or ester byproducts . For scale-up, recrystallization using solvent pairs (e.g., DMF/water) may enhance purity. Always compare NMR spectra (e.g., δ 4.09 ppm for ethyl protons) and HRMS data to confirm structural integrity .
Q. What spectroscopic techniques are critical for characterizing this compound, and how should data be interpreted?
Essential techniques include:
- <sup>1</sup>H NMR : Identify ethyl groups (quartet at δ 4.09 ppm, J = 7.1 Hz) and acetyl protons (singlet at δ 2.42 ppm) .
- <sup>13</sup>C NMR : Confirm carbonyl groups (δ 191.99 ppm for ketone, δ 172.51 ppm for ester) .
- HRMS : Verify molecular ion [M+H]<sup>+</sup> at m/z 248.1031 (C10H16BF2O4) for isotopic purity .
- IR : Detect C=O stretches (~1700–1750 cm<sup>-1</sup>) and ester C-O vibrations (~1200 cm<sup>-1</sup>).
Advanced Research Questions
Q. How does the equilibrium between keto-enol tautomers of this compound influence its reactivity in condensation reactions?
The keto-enol equilibrium (e.g., enols 4 and 5 ) governs nucleophilic attack pathways during pyrrole synthesis. For instance, condensation with 2-oximino-β-keto esters favors enol tautomers, directing acyl group loss and pyrrole formation (e.g., 9 vs. 10 ) . Use isotopic labeling (e.g., <sup>14</sup>C in acetoacetic esters) to quantify pathway dominance via specific activity comparisons (Table 1, ). Control pH and solvent polarity to stabilize reactive tautomers.
Q. What experimental strategies resolve contradictions in mechanistic data for this compound-derived pyrrole synthesis?
Discrepancies in pyrrole yields (e.g., 16.5% vs. higher yields in optimized protocols) may arise from competing pathways (e.g., acyl vs. ester group elimination). To resolve this:
- Isotopic tracing : Compare specific activities of starting esters and products to quantify pathway contributions .
- Kinetic studies : Monitor reaction progress via <sup>19</sup>F NMR or UPLC to identify rate-determining steps .
- Computational modeling : Calculate transition-state energies for competing mechanisms (e.g., DFT for tautomer stability).
Q. How can advanced synthetic methodologies (e.g., flow chemistry, microwave-assisted synthesis) improve the efficiency of this compound derivatization?
Flow reactors enhance reproducibility in BF3-catalyzed cyclizations by maintaining precise temperature/residence times . Microwave irradiation accelerates diketone-ester condensations, reducing side reactions. For stereoselective modifications, employ chiral auxiliaries or enzymes (e.g., lipases for ester hydrolysis).
Q. What role do computational tools (e.g., molecular docking, QSAR) play in predicting the bioactivity of this compound derivatives?
Molecular docking can model interactions with target enzymes (e.g., Plasmodium N-myristoyltransferases for antimalarial studies) . QSAR models correlate substituent effects (e.g., electron-withdrawing groups on the acetyl moiety) with bioactivity. Validate predictions via in vitro assays (e.g., IC50 measurements).
Q. How do steric and electronic factors influence the regioselectivity of nucleophilic attacks on this compound?
Steric hindrance at the 5-oxo group favors nucleophilic attack at the 4-acetyl position. Electronic effects (e.g., electron-deficient carbonyls) enhance electrophilicity at specific sites. Use Hammett plots to quantify substituent effects on reaction rates .
Q. What are the challenges in analyzing stereochemical outcomes of this compound reactions, and how can they be addressed?
Racemization during esterification or cyclization complicates stereochemical analysis. Employ chiral chromatography (e.g., Chiralpak columns) or Mosher ester derivatization to assign configurations. Dynamic NMR can detect enantiomerization barriers in solution.
Q. How should researchers troubleshoot low yields in multi-step syntheses involving this compound?
Common issues include:
- Intermediate instability : Protect sensitive groups (e.g., silyl ethers for hydroxyls).
- Byproduct formation : Optimize stoichiometry (e.g., 1:1 diketone:ester ratios) .
- Purification losses : Use traceless linkers or solid-phase synthesis for easier isolation.
Q. Methodological Best Practices
- Ethical considerations : Document synthetic protocols in detail (per IUPAC guidelines) to ensure reproducibility .
- Data reporting : Include raw NMR/UPLC datasets in supplementary materials .
- Statistical rigor : Apply t-tests or ANOVA to compare yields/pathway efficiencies .
Properties
IUPAC Name |
ethyl 4-acetyl-5-oxohexanoate | |
---|---|---|
Source | PubChem | |
URL | https://pubchem.ncbi.nlm.nih.gov | |
Description | Data deposited in or computed by PubChem | |
InChI |
InChI=1S/C10H16O4/c1-4-14-10(13)6-5-9(7(2)11)8(3)12/h9H,4-6H2,1-3H3 | |
Source | PubChem | |
URL | https://pubchem.ncbi.nlm.nih.gov | |
Description | Data deposited in or computed by PubChem | |
InChI Key |
YRSGDLIATOURQO-UHFFFAOYSA-N | |
Source | PubChem | |
URL | https://pubchem.ncbi.nlm.nih.gov | |
Description | Data deposited in or computed by PubChem | |
Canonical SMILES |
CCOC(=O)CCC(C(=O)C)C(=O)C | |
Source | PubChem | |
URL | https://pubchem.ncbi.nlm.nih.gov | |
Description | Data deposited in or computed by PubChem | |
Molecular Formula |
C10H16O4 | |
Source | PubChem | |
URL | https://pubchem.ncbi.nlm.nih.gov | |
Description | Data deposited in or computed by PubChem | |
DSSTOX Substance ID |
DTXSID30370046 | |
Record name | Ethyl 4-acetyl-5-oxohexanoate | |
Source | EPA DSSTox | |
URL | https://comptox.epa.gov/dashboard/DTXSID30370046 | |
Description | DSSTox provides a high quality public chemistry resource for supporting improved predictive toxicology. | |
Molecular Weight |
200.23 g/mol | |
Source | PubChem | |
URL | https://pubchem.ncbi.nlm.nih.gov | |
Description | Data deposited in or computed by PubChem | |
CAS No. |
2832-10-2 | |
Record name | Hexanoic acid, 4-acetyl-5-oxo-, ethyl ester | |
Source | CAS Common Chemistry | |
URL | https://commonchemistry.cas.org/detail?cas_rn=2832-10-2 | |
Description | CAS Common Chemistry is an open community resource for accessing chemical information. Nearly 500,000 chemical substances from CAS REGISTRY cover areas of community interest, including common and frequently regulated chemicals, and those relevant to high school and undergraduate chemistry classes. This chemical information, curated by our expert scientists, is provided in alignment with our mission as a division of the American Chemical Society. | |
Explanation | The data from CAS Common Chemistry is provided under a CC-BY-NC 4.0 license, unless otherwise stated. | |
Record name | Ethyl 4-acetyl-5-oxohexanoate | |
Source | EPA DSSTox | |
URL | https://comptox.epa.gov/dashboard/DTXSID30370046 | |
Description | DSSTox provides a high quality public chemistry resource for supporting improved predictive toxicology. | |
Record name | Hexanoic acid, 4-acetyl-5-oxo-, ethyl ester | |
Source | European Chemicals Agency (ECHA) | |
URL | https://echa.europa.eu/information-on-chemicals | |
Description | The European Chemicals Agency (ECHA) is an agency of the European Union which is the driving force among regulatory authorities in implementing the EU's groundbreaking chemicals legislation for the benefit of human health and the environment as well as for innovation and competitiveness. | |
Explanation | Use of the information, documents and data from the ECHA website is subject to the terms and conditions of this Legal Notice, and subject to other binding limitations provided for under applicable law, the information, documents and data made available on the ECHA website may be reproduced, distributed and/or used, totally or in part, for non-commercial purposes provided that ECHA is acknowledged as the source: "Source: European Chemicals Agency, http://echa.europa.eu/". Such acknowledgement must be included in each copy of the material. ECHA permits and encourages organisations and individuals to create links to the ECHA website under the following cumulative conditions: Links can only be made to webpages that provide a link to the Legal Notice page. | |
Synthesis routes and methods
Procedure details
Retrosynthesis Analysis
AI-Powered Synthesis Planning: Our tool employs the Template_relevance Pistachio, Template_relevance Bkms_metabolic, Template_relevance Pistachio_ringbreaker, Template_relevance Reaxys, Template_relevance Reaxys_biocatalysis model, leveraging a vast database of chemical reactions to predict feasible synthetic routes.
One-Step Synthesis Focus: Specifically designed for one-step synthesis, it provides concise and direct routes for your target compounds, streamlining the synthesis process.
Accurate Predictions: Utilizing the extensive PISTACHIO, BKMS_METABOLIC, PISTACHIO_RINGBREAKER, REAXYS, REAXYS_BIOCATALYSIS database, our tool offers high-accuracy predictions, reflecting the latest in chemical research and data.
Strategy Settings
Precursor scoring | Relevance Heuristic |
---|---|
Min. plausibility | 0.01 |
Model | Template_relevance |
Template Set | Pistachio/Bkms_metabolic/Pistachio_ringbreaker/Reaxys/Reaxys_biocatalysis |
Top-N result to add to graph | 6 |
Feasible Synthetic Routes
Disclaimer and Information on In-Vitro Research Products
Please be aware that all articles and product information presented on BenchChem are intended solely for informational purposes. The products available for purchase on BenchChem are specifically designed for in-vitro studies, which are conducted outside of living organisms. In-vitro studies, derived from the Latin term "in glass," involve experiments performed in controlled laboratory settings using cells or tissues. It is important to note that these products are not categorized as medicines or drugs, and they have not received approval from the FDA for the prevention, treatment, or cure of any medical condition, ailment, or disease. We must emphasize that any form of bodily introduction of these products into humans or animals is strictly prohibited by law. It is essential to adhere to these guidelines to ensure compliance with legal and ethical standards in research and experimentation.