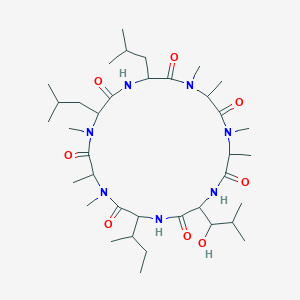
Ternatin heptapeptide
Overview
Description
Ternatin is a naturally occurring cyclic peptide produced by several species of fungiTernatin is known for its ability to inhibit protein synthesis by targeting the elongation factor-1A ternary complex, making it a promising candidate for therapeutic development .
Mechanism of Action
Target of Action
Ternatin heptapeptide, also known as Ternatin, primarily targets the translation elongation factor-1A ternary complex (eEF1A·GTP·aminoacyl-tRNA) . This complex plays a crucial role in protein synthesis, a central biological process .
Mode of Action
Ternatin disrupts protein translation by binding to the eEF1A ternary complex . It competes with other natural products, such as didemnin and cytotrienin, for binding to this complex . Mutations in domain III of eEF1A can prevent Ternatin binding and confer resistance to its cytotoxic effects .
Biochemical Pathways
The primary biochemical pathway affected by Ternatin is the protein translation pathway . eEF1A, the target of Ternatin, participates in a series of reactions with guanine nucleotides, transfer RNAs (tRNAs), a guanine nucleotide exchange factor, and the elongating ribosome . By binding to the eEF1A ternary complex, Ternatin disrupts these reactions and inhibits protein synthesis .
Pharmacokinetics
It’s known that ternatin and its synthetic variants can penetrate cells and exhibit cytotoxic activity . More research is needed to fully understand the ADME properties of Ternatin and their impact on its bioavailability.
Result of Action
Ternatin and its synthetic variants can kill cancer cells by targeting the eEF1A ternary complex . The synthetic variants of Ternatin have shown up to 500-fold greater potency than Ternatin itself . Furthermore, Ternatin has been found to suppress hyperglycemia and hepatic fatty acid synthesis in spontaneously diabetic mice .
Biochemical Analysis
Biochemical Properties
Ternatin heptapeptide disrupts a central biological process, protein translation, by binding to the translation elongation factor 1A . This protein participates in a carefully orchestrated series of reactions with guanine nucleotides, transfer RNAs (tRNAs), a guanine nucleotide exchange factor, and the elongating ribosome . The nature of these interactions is complex and involves a multitude of biomolecules.
Cellular Effects
This compound has been found to have significant effects on various types of cells. It is cytotoxic toward cancer cells, with synthetic variants showing up to 500-fold greater potency than Ternatin itself . It inhibits protein synthesis in cells, which correlates with its ability to block cell proliferation .
Molecular Mechanism
The molecular mechanism of action of this compound involves its binding to the translation elongation factor-1A ternary complex (eEF1A·GTP·aminoacyl-tRNA) as a specific target . Mutations in domain III of eEF1A prevent Ternatin binding and confer resistance to its cytotoxic effects, implicating the adjacent hydrophobic surface as a functional hot spot for eEF1A modulation .
Temporal Effects in Laboratory Settings
It has been observed that Ternatin can kill mammalian cells in high doses
Dosage Effects in Animal Models
The effects of this compound at different dosages in animal models have not been extensively studied. It is known that Ternatin can kill mammalian cells in high doses
Metabolic Pathways
This compound is involved in the protein translation pathway, specifically by binding to the translation elongation factor 1A This interaction disrupts the normal function of this pathway
Transport and Distribution
Given its role in protein translation, it is likely that it interacts with various transporters and binding proteins involved in this process .
Subcellular Localization
Given its role in protein translation, it is likely that it is localized to the ribosomes where protein synthesis occurs
Preparation Methods
Synthetic Routes and Reaction Conditions: Ternatin and its synthetic variants can be prepared through solid-phase peptide synthesis (SPPS). This method involves the sequential addition of amino acids to a growing peptide chain anchored to a solid resin. The process includes deprotection and coupling steps, which are repeated until the desired peptide sequence is obtained. The final product is then cleaved from the resin and purified .
Industrial Production Methods: Industrial production of ternatin involves fermentation processes using fungi that naturally produce the compound. The fungi are cultured in large bioreactors under controlled conditions to optimize the yield of ternatin. The compound is then extracted and purified using chromatographic techniques .
Chemical Reactions Analysis
Types of Reactions: Ternatin undergoes various chemical reactions, including:
Oxidation: Ternatin can be oxidized to form different derivatives with altered biological activities.
Reduction: Reduction reactions can modify the peptide bonds within ternatin, potentially affecting its stability and activity.
Substitution: Substitution reactions can introduce new functional groups into the ternatin molecule, enhancing its properties.
Common Reagents and Conditions:
Oxidation: Common oxidizing agents include hydrogen peroxide and potassium permanganate.
Reduction: Reducing agents such as sodium borohydride and lithium aluminum hydride are used.
Substitution: Reagents like alkyl halides and acyl chlorides are employed for substitution reactions.
Major Products Formed: The major products formed from these reactions include various ternatin derivatives with enhanced cytotoxicity and improved pharmacokinetic properties .
Scientific Research Applications
Ternatin has a wide range of scientific research applications, including:
Chemistry: Ternatin serves as a valuable chemical probe for studying protein synthesis and translation elongation.
Biology: It is used to investigate the mechanisms of protein translation and the role of elongation factor-1A in cellular processes.
Medicine: Ternatin and its derivatives are being explored as potential anticancer agents due to their ability to inhibit protein synthesis in cancer cells.
Industry: Ternatin’s unique properties make it a candidate for developing new therapeutic drugs and chemical probes
Comparison with Similar Compounds
Didemnin: Another cyclic peptide that targets the elongation factor-1A ternary complex but exhibits different kinetic properties.
Cytotrienin: A natural product that also binds to eEF1A and inhibits protein synthesis.
Uniqueness of Ternatin: Ternatin is unique due to its specific binding affinity for the eEF1A ternary complex and its ability to induce degradation of eEF1A. This degradation is not observed with other similar compounds like didemnin, making ternatin a distinct and valuable compound for therapeutic development .
Properties
IUPAC Name |
(3S,6S,9S,12S,15R,18R,21R)-15-[(2S)-butan-2-yl]-18-[(1R)-1-hydroxy-2-methylpropyl]-1,3,4,10,12,13,21-heptamethyl-6,9-bis(2-methylpropyl)-1,4,7,10,13,16,19-heptazacyclohenicosane-2,5,8,11,14,17,20-heptone | |
---|---|---|
Details | Computed by LexiChem 2.6.6 (PubChem release 2019.06.18) | |
Source | PubChem | |
URL | https://pubchem.ncbi.nlm.nih.gov | |
Description | Data deposited in or computed by PubChem | |
InChI |
InChI=1S/C37H67N7O8/c1-16-22(8)28-37(52)43(14)25(11)35(50)44(15)27(18-20(4)5)32(47)38-26(17-19(2)3)36(51)42(13)24(10)34(49)41(12)23(9)31(46)40-29(33(48)39-28)30(45)21(6)7/h19-30,45H,16-18H2,1-15H3,(H,38,47)(H,39,48)(H,40,46)/t22-,23+,24-,25-,26-,27-,28+,29+,30+/m0/s1 | |
Details | Computed by InChI 1.0.5 (PubChem release 2019.06.18) | |
Source | PubChem | |
URL | https://pubchem.ncbi.nlm.nih.gov | |
Description | Data deposited in or computed by PubChem | |
InChI Key |
ZMFVAIFXJWEOMH-PTPSPKLBSA-N | |
Details | Computed by InChI 1.0.5 (PubChem release 2019.06.18) | |
Source | PubChem | |
URL | https://pubchem.ncbi.nlm.nih.gov | |
Description | Data deposited in or computed by PubChem | |
Canonical SMILES |
CCC(C)C1C(=O)N(C(C(=O)N(C(C(=O)NC(C(=O)N(C(C(=O)N(C(C(=O)NC(C(=O)N1)C(C(C)C)O)C)C)C)C)CC(C)C)CC(C)C)C)C)C | |
Details | Computed by OEChem 2.1.5 (PubChem release 2019.06.18) | |
Source | PubChem | |
URL | https://pubchem.ncbi.nlm.nih.gov | |
Description | Data deposited in or computed by PubChem | |
Isomeric SMILES |
CC[C@H](C)[C@@H]1C(=O)N([C@H](C(=O)N([C@H](C(=O)N[C@H](C(=O)N([C@H](C(=O)N([C@@H](C(=O)N[C@@H](C(=O)N1)[C@@H](C(C)C)O)C)C)C)C)CC(C)C)CC(C)C)C)C)C | |
Details | Computed by OEChem 2.1.5 (PubChem release 2019.06.18) | |
Source | PubChem | |
URL | https://pubchem.ncbi.nlm.nih.gov | |
Description | Data deposited in or computed by PubChem | |
Molecular Formula |
C37H67N7O8 | |
Details | Computed by PubChem 2.1 (PubChem release 2019.06.18) | |
Source | PubChem | |
URL | https://pubchem.ncbi.nlm.nih.gov | |
Description | Data deposited in or computed by PubChem | |
DSSTOX Substance ID |
DTXSID20933442 | |
Record name | Ternatin | |
Source | EPA DSSTox | |
URL | https://comptox.epa.gov/dashboard/DTXSID20933442 | |
Description | DSSTox provides a high quality public chemistry resource for supporting improved predictive toxicology. | |
Molecular Weight |
738.0 g/mol | |
Details | Computed by PubChem 2.1 (PubChem release 2021.05.07) | |
Source | PubChem | |
URL | https://pubchem.ncbi.nlm.nih.gov | |
Description | Data deposited in or computed by PubChem | |
CAS No. |
148619-41-4 | |
Record name | Ternatin | |
Source | EPA DSSTox | |
URL | https://comptox.epa.gov/dashboard/DTXSID20933442 | |
Description | DSSTox provides a high quality public chemistry resource for supporting improved predictive toxicology. | |
Retrosynthesis Analysis
AI-Powered Synthesis Planning: Our tool employs the Template_relevance Pistachio, Template_relevance Bkms_metabolic, Template_relevance Pistachio_ringbreaker, Template_relevance Reaxys, Template_relevance Reaxys_biocatalysis model, leveraging a vast database of chemical reactions to predict feasible synthetic routes.
One-Step Synthesis Focus: Specifically designed for one-step synthesis, it provides concise and direct routes for your target compounds, streamlining the synthesis process.
Accurate Predictions: Utilizing the extensive PISTACHIO, BKMS_METABOLIC, PISTACHIO_RINGBREAKER, REAXYS, REAXYS_BIOCATALYSIS database, our tool offers high-accuracy predictions, reflecting the latest in chemical research and data.
Strategy Settings
Precursor scoring | Relevance Heuristic |
---|---|
Min. plausibility | 0.01 |
Model | Template_relevance |
Template Set | Pistachio/Bkms_metabolic/Pistachio_ringbreaker/Reaxys/Reaxys_biocatalysis |
Top-N result to add to graph | 6 |
Feasible Synthetic Routes
A: Ternatin targets the eukaryotic elongation factor-1A (eEF1A) ternary complex, specifically the eEF1A·GTP·aminoacyl-tRNA complex. [, , ] This complex plays a crucial role in protein synthesis by delivering aminoacyl-tRNAs to the ribosome.
A: Ternatin binding traps eEF1A in an intermediate state of aminoacyl-tRNA selection, preventing its release and subsequent accommodation of the aminoacyl-tRNA into the ribosome. [, ] This effectively halts protein translation.
A: Yes, studies have shown that ternatin induces the degradation of eEF1A, a phenomenon not observed with other eEF1A inhibitors like didemnin B. [, ] This degradation appears to involve a ubiquitin-dependent pathway and may be linked to ribosome-associated quality control mechanisms.
A: By inhibiting eEF1A and disrupting protein synthesis, ternatin exhibits cytotoxic effects, particularly against cancer cells. [, ] This has sparked interest in its potential as an anticancer agent.
A: Ternatin is a cyclic heptapeptide. Its molecular formula is C38H65N7O9 and its molecular weight is 763.96 g/mol. []
A: The presence of four N-methylated amino acid residues in ternatin's structure appears to restrict the number of low-energy conformations the molecule can adopt. [] This conformational rigidity may contribute to its specific biological activity.
A: Ternatin is characterized by a beta-turn structure stabilized by intramolecular hydrogen bonds. [] This structural feature has been shown to be essential for its fat-accumulation inhibitory activity.
ANone: The provided research focuses on ternatin's biological activity and does not offer insights into material compatibility or stability relevant for material science applications.
ANone: Ternatin is not reported to have catalytic properties. Its mechanism of action primarily involves binding and inhibiting a biological target, eEF1A, rather than catalyzing a chemical reaction.
A: Yes, molecular docking simulations have been employed to explore ternatin's interactions with potential targets, including cholera toxin and SARS-CoV-2 nucleocapsid protein. [, ] These studies provide insights into ternatin's binding affinities and potential therapeutic applications.
A: While the provided research does not mention specific QSAR studies on ternatin, one study utilized molecular modeling to analyze ternatin's electronic and structural characteristics, laying the groundwork for future drug design and QSAR investigations. []
A: Studies focusing on the beta-OH-D-Leu moiety of ternatin have demonstrated the importance of its backbone conformation for fat-accumulation inhibitory activity. [, ] Modifications that disrupt this conformation could potentially diminish its efficacy.
A: Systematic replacement of each amino acid in ternatin with alanine revealed that the D-Ile residue plays a crucial role in its biological activity. [] This finding highlights the importance of specific amino acid residues in maintaining ternatin's potency.
Disclaimer and Information on In-Vitro Research Products
Please be aware that all articles and product information presented on BenchChem are intended solely for informational purposes. The products available for purchase on BenchChem are specifically designed for in-vitro studies, which are conducted outside of living organisms. In-vitro studies, derived from the Latin term "in glass," involve experiments performed in controlled laboratory settings using cells or tissues. It is important to note that these products are not categorized as medicines or drugs, and they have not received approval from the FDA for the prevention, treatment, or cure of any medical condition, ailment, or disease. We must emphasize that any form of bodily introduction of these products into humans or animals is strictly prohibited by law. It is essential to adhere to these guidelines to ensure compliance with legal and ethical standards in research and experimentation.