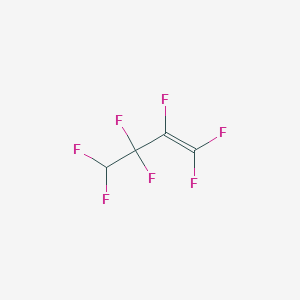
1,1,2,3,3,4,4-Heptafluorobut-1-ene
Overview
Description
1,1,2,3,3,4,4-Heptafluorobut-1-ene is a fluorinated olefin with the molecular formula C4HF7 This compound is characterized by the presence of seven fluorine atoms attached to a butene backbone It is a colorless gas at room temperature and is known for its high reactivity due to the presence of multiple fluorine atoms
Preparation Methods
Synthetic Routes and Reaction Conditions
1,1,2,3,3,4,4-Heptafluorobut-1-ene can be synthesized through the fluorination of butene derivatives. One common method involves the reaction of butene with fluorine gas in the presence of a catalyst. The reaction is typically carried out under controlled conditions to ensure the selective addition of fluorine atoms to the butene backbone.
Industrial Production Methods
In industrial settings, the production of this compound often involves the use of specialized reactors designed to handle the highly reactive fluorine gas. The process may include multiple steps, such as the initial fluorination of butene followed by purification and separation of the desired product. The use of advanced materials and technologies is essential to ensure the safety and efficiency of the production process.
Chemical Reactions Analysis
Types of Reactions
1,1,2,3,3,4,4-Heptafluorobut-1-ene undergoes various chemical reactions, including:
Substitution: Fluorinated olefins like this compound can undergo substitution reactions where one or more fluorine atoms are replaced by other atoms or groups.
Common Reagents and Conditions
Oxidizing Agents: Chlorine atoms are commonly used to initiate the oxidation of this compound.
Catalysts: Catalysts such as metal fluorides may be used to facilitate the fluorination reactions.
Major Products Formed
Carbonyl Difluoride: Formed during the oxidation of this compound.
Tetrafluoropropanoyl Fluoride: Another major product of the oxidation reaction.
Scientific Research Applications
1,1,2,3,3,4,4-Heptafluorobut-1-ene has several scientific research applications:
Atmospheric Chemistry: The compound is studied for its potential impact on atmospheric chemistry and its role in mitigating climate change due to its low global warming potential.
Materials Science: It is used in the development of advanced materials, including fluorinated polymers and coatings.
Industrial Applications: The compound is explored for use in various industrial processes, including the production of specialty chemicals and refrigerants.
Mechanism of Action
The mechanism of action of 1,1,2,3,3,4,4-Heptafluorobut-1-ene involves its high reactivity due to the presence of multiple fluorine atoms. The compound can readily participate in radical reactions, where the addition of radicals such as chlorine atoms leads to the formation of reactive intermediates. These intermediates can further react to form stable products such as carbonyl difluoride and tetrafluoropropanoyl fluoride . The molecular targets and pathways involved in these reactions are primarily related to the formation and stabilization of radical species.
Comparison with Similar Compounds
1,1,2,3,3,4,4-Heptafluorobut-1-ene can be compared with other fluorinated olefins, such as:
1,1,1,3,4,4,4-Heptafluorobut-2-ene: Another fluorinated olefin with similar properties but different structural arrangement.
Tetrafluoroethylene: A simpler fluorinated olefin with fewer fluorine atoms, used in the production of polytetrafluoroethylene (PTFE).
The uniqueness of this compound lies in its specific arrangement of fluorine atoms, which imparts distinct reactivity and properties compared to other fluorinated olefins.
Properties
IUPAC Name |
1,1,2,3,3,4,4-heptafluorobut-1-ene | |
---|---|---|
Source | PubChem | |
URL | https://pubchem.ncbi.nlm.nih.gov | |
Description | Data deposited in or computed by PubChem | |
InChI |
InChI=1S/C4HF7/c5-1(2(6)7)4(10,11)3(8)9/h3H | |
Source | PubChem | |
URL | https://pubchem.ncbi.nlm.nih.gov | |
Description | Data deposited in or computed by PubChem | |
InChI Key |
NUPBXTZOBYEVIR-UHFFFAOYSA-N | |
Source | PubChem | |
URL | https://pubchem.ncbi.nlm.nih.gov | |
Description | Data deposited in or computed by PubChem | |
Canonical SMILES |
C(C(C(=C(F)F)F)(F)F)(F)F | |
Source | PubChem | |
URL | https://pubchem.ncbi.nlm.nih.gov | |
Description | Data deposited in or computed by PubChem | |
Molecular Formula |
C4HF7 | |
Source | PubChem | |
URL | https://pubchem.ncbi.nlm.nih.gov | |
Description | Data deposited in or computed by PubChem | |
DSSTOX Substance ID |
DTXSID50379253 | |
Record name | 4H-Perfluoro-1-butene | |
Source | EPA DSSTox | |
URL | https://comptox.epa.gov/dashboard/DTXSID50379253 | |
Description | DSSTox provides a high quality public chemistry resource for supporting improved predictive toxicology. | |
Molecular Weight |
182.04 g/mol | |
Source | PubChem | |
URL | https://pubchem.ncbi.nlm.nih.gov | |
Description | Data deposited in or computed by PubChem | |
CAS No. |
680-54-6 | |
Record name | 4H-Perfluoro-1-butene | |
Source | EPA DSSTox | |
URL | https://comptox.epa.gov/dashboard/DTXSID50379253 | |
Description | DSSTox provides a high quality public chemistry resource for supporting improved predictive toxicology. | |
Retrosynthesis Analysis
AI-Powered Synthesis Planning: Our tool employs the Template_relevance Pistachio, Template_relevance Bkms_metabolic, Template_relevance Pistachio_ringbreaker, Template_relevance Reaxys, Template_relevance Reaxys_biocatalysis model, leveraging a vast database of chemical reactions to predict feasible synthetic routes.
One-Step Synthesis Focus: Specifically designed for one-step synthesis, it provides concise and direct routes for your target compounds, streamlining the synthesis process.
Accurate Predictions: Utilizing the extensive PISTACHIO, BKMS_METABOLIC, PISTACHIO_RINGBREAKER, REAXYS, REAXYS_BIOCATALYSIS database, our tool offers high-accuracy predictions, reflecting the latest in chemical research and data.
Strategy Settings
Precursor scoring | Relevance Heuristic |
---|---|
Min. plausibility | 0.01 |
Model | Template_relevance |
Template Set | Pistachio/Bkms_metabolic/Pistachio_ringbreaker/Reaxys/Reaxys_biocatalysis |
Top-N result to add to graph | 6 |
Feasible Synthetic Routes
Q1: How does 1,1,2,3,3,4,4-Heptafluorobut-1-ene interact with chlorine atoms in the atmosphere, and what are the environmental consequences?
A1: The study investigates the reaction of this compound with chlorine atoms, a process relevant to atmospheric chemistry. The research reveals that this reaction primarily generates two main products: carbonyl difluoride (CF2=O) and 2,2,3,3-tetrafluoropropanoyl fluoride (O=CFCF2CF2H) []. Importantly, the study determined the Global Warming Potentials (GWPs) of these products to be low, indicating a minimal contribution to climate change compared to other greenhouse gases.
Q2: What spectroscopic data was used to characterize this compound and its reaction products?
A2: The research employed Fourier-transform infrared spectroscopy (FTIR) to monitor the reaction and identify the products []. Additionally, the study utilized density functional theory (DFT) calculations to predict the anharmonic vibrational frequencies of this compound and 2,2,3,3-tetrafluoropropanoyl fluoride, taking into account various low-energy conformations. These calculated frequencies showed good agreement with the experimental FTIR measurements, confirming the product identification and providing insights into their molecular structures.
Disclaimer and Information on In-Vitro Research Products
Please be aware that all articles and product information presented on BenchChem are intended solely for informational purposes. The products available for purchase on BenchChem are specifically designed for in-vitro studies, which are conducted outside of living organisms. In-vitro studies, derived from the Latin term "in glass," involve experiments performed in controlled laboratory settings using cells or tissues. It is important to note that these products are not categorized as medicines or drugs, and they have not received approval from the FDA for the prevention, treatment, or cure of any medical condition, ailment, or disease. We must emphasize that any form of bodily introduction of these products into humans or animals is strictly prohibited by law. It is essential to adhere to these guidelines to ensure compliance with legal and ethical standards in research and experimentation.