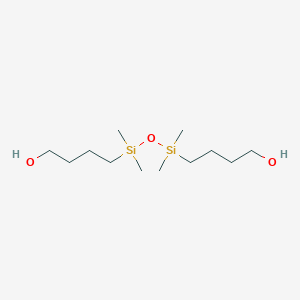
1,3-Bis(4-hydroxybutyl)tetramethyldisiloxane
Overview
Description
1,3-Bis(4-hydroxybutyl)tetramethyldisiloxane is a versatile chemical compound with the molecular formula C12H30O3Si2. It is a colorless to almost colorless clear liquid with a mild odor. This compound is widely used in various industries due to its unique properties, including its role as a coupling agent, surface modifier, and cross-linking agent .
Preparation Methods
Synthetic Routes and Reaction Conditions
1,3-Bis(4-hydroxybutyl)tetramethyldisiloxane can be synthesized through a two-step process:
Industrial Production Methods
Industrial production of this compound typically involves the same synthetic route but on a larger scale, with optimized reaction conditions to ensure high yield and purity. The compound is often produced in facilities equipped with advanced chemical processing equipment to handle the reagents and reaction conditions safely and efficiently .
Chemical Reactions Analysis
Types of Reactions
1,3-Bis(4-hydroxybutyl)tetramethyldisiloxane undergoes various chemical reactions, including:
Oxidation: The hydroxyl groups can be oxidized to form corresponding carbonyl compounds.
Substitution: The hydroxyl groups can participate in substitution reactions to form ethers or esters.
Polymerization: It can act as a monomer in the formation of polysiloxanes and other silicon-based polymers.
Common Reagents and Conditions
Oxidation: Common oxidizing agents like potassium permanganate or chromium trioxide can be used.
Substitution: Reagents such as alkyl halides or acyl chlorides in the presence of a base can facilitate substitution reactions.
Polymerization: Catalysts like platinum or tin compounds are often used to initiate polymerization reactions.
Major Products
Oxidation: Formation of carbonyl compounds such as aldehydes or ketones.
Substitution: Formation of ethers or esters depending on the substituent used.
Polymerization: Formation of polysiloxanes and other silicon-based polymers.
Scientific Research Applications
1,3-Bis(4-hydroxybutyl)tetramethyldisiloxane has a wide range of applications in scientific research and industry:
Chemistry: Used as a coupling agent and cross-linking agent in the synthesis of various polymers and materials.
Biology: Employed in the modification of surfaces for biological assays and experiments.
Industry: Added to lubricants, sealants, and adhesives to enhance flexibility, adhesion, and wear resistance.
Mechanism of Action
The mechanism of action of 1,3-Bis(4-hydroxybutyl)tetramethyldisiloxane involves its ability to form strong bonds with various substrates through its hydroxyl groups. These hydroxyl groups can undergo condensation reactions with other functional groups, leading to the formation of stable linkages. This property makes it an effective coupling agent and cross-linking agent in various applications .
Comparison with Similar Compounds
Similar Compounds
Uniqueness
1,3-Bis(4-hydroxybutyl)tetramethyldisiloxane is unique due to its dual hydroxyl functionality, which allows it to participate in a wide range of chemical reactions. This dual functionality also enhances its effectiveness as a coupling agent and cross-linking agent compared to similar compounds that may lack one or both hydroxyl groups .
Properties
IUPAC Name |
4-[[4-hydroxybutyl(dimethyl)silyl]oxy-dimethylsilyl]butan-1-ol | |
---|---|---|
Source | PubChem | |
URL | https://pubchem.ncbi.nlm.nih.gov | |
Description | Data deposited in or computed by PubChem | |
InChI |
InChI=1S/C12H30O3Si2/c1-16(2,11-7-5-9-13)15-17(3,4)12-8-6-10-14/h13-14H,5-12H2,1-4H3 | |
Source | PubChem | |
URL | https://pubchem.ncbi.nlm.nih.gov | |
Description | Data deposited in or computed by PubChem | |
InChI Key |
OWJKJLOCIDNNGJ-UHFFFAOYSA-N | |
Source | PubChem | |
URL | https://pubchem.ncbi.nlm.nih.gov | |
Description | Data deposited in or computed by PubChem | |
Canonical SMILES |
C[Si](C)(CCCCO)O[Si](C)(C)CCCCO | |
Source | PubChem | |
URL | https://pubchem.ncbi.nlm.nih.gov | |
Description | Data deposited in or computed by PubChem | |
Molecular Formula |
C12H30O3Si2 | |
Source | PubChem | |
URL | https://pubchem.ncbi.nlm.nih.gov | |
Description | Data deposited in or computed by PubChem | |
DSSTOX Substance ID |
DTXSID80377681 | |
Record name | 1,3-Bis(4-hydroxybutyl)tetramethyldisiloxane | |
Source | EPA DSSTox | |
URL | https://comptox.epa.gov/dashboard/DTXSID80377681 | |
Description | DSSTox provides a high quality public chemistry resource for supporting improved predictive toxicology. | |
Molecular Weight |
278.53 g/mol | |
Source | PubChem | |
URL | https://pubchem.ncbi.nlm.nih.gov | |
Description | Data deposited in or computed by PubChem | |
CAS No. |
5931-17-9 | |
Record name | 1,3-Bis(4-hydroxybutyl)tetramethyldisiloxane | |
Source | CAS Common Chemistry | |
URL | https://commonchemistry.cas.org/detail?cas_rn=5931-17-9 | |
Description | CAS Common Chemistry is an open community resource for accessing chemical information. Nearly 500,000 chemical substances from CAS REGISTRY cover areas of community interest, including common and frequently regulated chemicals, and those relevant to high school and undergraduate chemistry classes. This chemical information, curated by our expert scientists, is provided in alignment with our mission as a division of the American Chemical Society. | |
Explanation | The data from CAS Common Chemistry is provided under a CC-BY-NC 4.0 license, unless otherwise stated. | |
Record name | 1,3-Bis(4-hydroxybutyl)tetramethyldisiloxane | |
Source | EPA DSSTox | |
URL | https://comptox.epa.gov/dashboard/DTXSID80377681 | |
Description | DSSTox provides a high quality public chemistry resource for supporting improved predictive toxicology. | |
Retrosynthesis Analysis
AI-Powered Synthesis Planning: Our tool employs the Template_relevance Pistachio, Template_relevance Bkms_metabolic, Template_relevance Pistachio_ringbreaker, Template_relevance Reaxys, Template_relevance Reaxys_biocatalysis model, leveraging a vast database of chemical reactions to predict feasible synthetic routes.
One-Step Synthesis Focus: Specifically designed for one-step synthesis, it provides concise and direct routes for your target compounds, streamlining the synthesis process.
Accurate Predictions: Utilizing the extensive PISTACHIO, BKMS_METABOLIC, PISTACHIO_RINGBREAKER, REAXYS, REAXYS_BIOCATALYSIS database, our tool offers high-accuracy predictions, reflecting the latest in chemical research and data.
Strategy Settings
Precursor scoring | Relevance Heuristic |
---|---|
Min. plausibility | 0.01 |
Model | Template_relevance |
Template Set | Pistachio/Bkms_metabolic/Pistachio_ringbreaker/Reaxys/Reaxys_biocatalysis |
Top-N result to add to graph | 6 |
Feasible Synthetic Routes
Q1: How does 1,3-Bis(4-hydroxybutyl)tetramethyldisiloxane (BHTD) impact the properties of polyurethane elastomers?
A: BHTD acts as a secondary chain extender in polyurethane elastomers, influencing both their mechanical properties and morphology. Research indicates that incorporating BHTD can disrupt the order of hard segments within the polymer [, ]. This disruption generally leads to elastomers with a lower flexural modulus and increased elongation, desirable traits for flexible materials.
Q2: Are there differences in how BHTD behaves compared to other chain extenders?
A: Yes, studies have shown that compared to non-silicon-based chain extenders like 2,2,3,3,4,4-hexafluoro-1,5-pentanediol (HFPD), BHTD causes less disruption to the hard segment order in polyurethane elastomers []. This difference is particularly noticeable when BHTD is used in lower concentrations. Additionally, BHTD, being silicon-based, improves the compatibility of polydimethylsiloxane (PDMS) soft segments with the harder segments of the polyurethane. This enhanced compatibility is not observed with chain extenders like HFPD and hydroquinone bis(2-hydroxyethyl)ether (HQHE) [].
Q3: How is this compound (BHTD) synthesized?
A: BHTD can be directly synthesized using a relatively straightforward method. This involves reacting dimethyldichlorosilane with purified tetrahydrofuran and magnesium powder in the presence of ethyl iodide and iodine as catalysts []. This reaction yields the desired BHTD molecule.
Q4: What are the characterization techniques used to confirm the successful synthesis of BHTD?
A: Researchers primarily utilize Fourier transform infrared (FTIR) spectroscopy and high-resolution 1H NMR to confirm the successful synthesis of BHTD []. These techniques provide detailed information about the molecule's structure, confirming the presence of the desired functional groups and bonding patterns.
Disclaimer and Information on In-Vitro Research Products
Please be aware that all articles and product information presented on BenchChem are intended solely for informational purposes. The products available for purchase on BenchChem are specifically designed for in-vitro studies, which are conducted outside of living organisms. In-vitro studies, derived from the Latin term "in glass," involve experiments performed in controlled laboratory settings using cells or tissues. It is important to note that these products are not categorized as medicines or drugs, and they have not received approval from the FDA for the prevention, treatment, or cure of any medical condition, ailment, or disease. We must emphasize that any form of bodily introduction of these products into humans or animals is strictly prohibited by law. It is essential to adhere to these guidelines to ensure compliance with legal and ethical standards in research and experimentation.