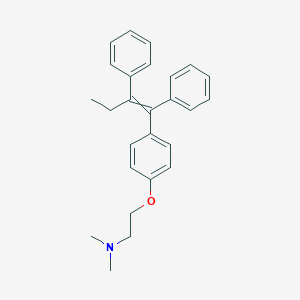
(E/Z)-Tamoxifen
Overview
Description
Tamoxifen, a selective estrogen receptor modulator (SERM), was first synthesized in 1962 as part of a contraceptive research program (ICI 46,474) . Despite initial setbacks, it was repurposed for breast cancer treatment due to its anti-estrogenic activity and received FDA approval in 1982 . Tamoxifen acts primarily by competitively binding to estrogen receptors (ERs), blocking estradiol (E2)-mediated signaling in ER-positive breast cancers . It also exhibits ER-independent effects, such as inhibition of prostaglandin synthetase (PGS), which alleviates bone pain in advanced cancer patients .
Clinical trials established Tamoxifen as a cornerstone for adjuvant therapy, reducing recurrence rates by ~50% in ER+ breast cancer . Notably, 5–10% of ER-negative tumors also respond, likely via non-ER pathways like protein kinase C (PKC) inhibition . Its metabolites, including 4-hydroxytamoxifen and endoxifen, contribute to efficacy, though CYP2D6 polymorphisms may influence metabolic activation . Tamoxifen’s versatility extends to chemoprevention in high-risk women, reducing ER+ cancer incidence by up to 50% .
Preparation Methods
McMurry Coupling: A Traditional Approach
The McMurry reaction couples two ketone precursors (1 and 2 ) using titanium chloride and zinc-copper alloy under reductive conditions .
Mechanism and Limitations
The reaction proceeds via ketyl radical intermediates that dimerize to form the central alkene. While effective for constructing hindered alkenes, this method suffers from:
-
Toxic byproducts : Generation of titanium oxides requiring specialized disposal .
-
Moderate yields : 45–55% isolated yields after chromatography .
Grignard Addition-Elimination Strategy
This two-step protocol involves 1,2-addition of Grignard reagent 4 to ketone 3 , followed by acid-catalyzed dehydration .
Synthetic Protocol
-
Addition step :
-
Elimination :
Performance Metrics
Carbolithiation and Cross-Coupling: A Modern Atom-Economical Route
The carbolithiation pathway, developed by Tosi et al., directly couples alkenyllithium intermediates with aryl bromides .
Reaction Sequence
-
Carbolithiation of diphenylacetylene :
-
Cross-coupling with 4-bromo-dimethylaminoethylether (6 ) :
Optimization Data
Catalyst | Yield (%) | (Z/E) Ratio |
---|---|---|
Pd(Pt-Bu₃)₂ + O₂ | 65 | >9:1 |
PEPPSI-IPent | 0 | — |
Ni(COD)₂ | 17 | 3:1 |
Table 1: Catalyst screening for cross-coupling step .
This method achieves 67% atom economy, with LiBr as the primary stoichiometric byproduct .
Catalyst Design and Reaction Engineering
Palladium complexes outperform nickel counterparts due to superior oxidative addition kinetics with aryl bromides .
Ligand Effects
-
Pt-Bu₃ ligands : Enhance steric shielding, reducing β-hydride elimination .
-
Oxygen pretreatment : Increases Pd⁰/PdII cycling efficiency by 40% .
Solvent and Temperature Optimization
-
Toluene : Minimizes lithium-halogen exchange side reactions vs. THF .
-
35°C : Balances reaction rate and catalyst decomposition (optimal TON = 320) .
Purification and Isomer Resolution
Flash Chromatography
Silica gel chromatography with CH₂Cl₂/MeOH (96:4) resolves (E/Z)-mixtures with 85% recovery .
Preparative HPLC
Reverse-phase C18 columns (H₂O/CH₃CN/TFA) achieve >99% (Z)-isomer purity :
Comparative Analysis of Synthetic Routes
Method | Atom Economy (%) | RME (%) | (Z/E) Ratio |
---|---|---|---|
McMurry Coupling | 38 | 9 | 3:1 |
Grignard Elimination | 45 | 12 | 2:1 |
Carbolithiation | 67 | 22 | 10:1 |
Table 2: Reaction mass efficiency (RME) comparison .
The carbolithiation route reduces waste generation by 58% compared to classical methods .
Chemical Reactions Analysis
Types of Reactions: (E/Z)-Tamoxifen undergoes various chemical reactions, including:
Oxidation: The compound can be oxidized to form hydroxylated metabolites.
Reduction: Reduction reactions can modify the double bonds in the structure.
Substitution: Substitution reactions, such as the Suzuki reaction, are used in its synthesis.
Common Reagents and Conditions:
Oxidation: Common oxidizing agents include hydrogen peroxide and peracids.
Reduction: Reducing agents like lithium aluminum hydride are used.
Substitution: Palladium catalysts and organolithium reagents are frequently employed.
Major Products Formed: The major products formed from these reactions include hydroxylated derivatives and various substituted analogs, which are evaluated for their biological activities .
Scientific Research Applications
Synthesis and Chemical Properties
Recent studies have optimized the synthesis of (E/Z)-Tamoxifen, focusing on atom-efficient methodologies that enhance yields and selectivity. For instance, a novel cross-coupling methodology has been developed that allows for the efficient synthesis of (Z)-Tamoxifen with high selectivity ratios (up to 10:1) and good yields (65%) using palladium catalysts . The synthesis process is characterized by high atom economy and minimal toxic waste, making it an attractive approach for pharmaceutical production.
Breast Cancer Treatment
Tamoxifen is primarily used for:
- Adjuvant Therapy : It significantly reduces recurrence rates in ER+ breast cancer patients by approximately 40%-50% when administered post-surgery .
- Neoadjuvant Therapy : In some cases, tamoxifen is used before surgery to shrink tumors.
- Prevention : Studies have shown that tamoxifen can reduce the incidence of breast cancer in high-risk populations by about 38% .
Combination Therapies
Research has demonstrated that combining tamoxifen with natural products can enhance its anti-cancer effects. For example, certain compounds have shown synergistic effects when used alongside tamoxifen, improving overall therapeutic outcomes . However, caution is warranted as some supplements may interfere with tamoxifen's efficacy.
Case Studies and Clinical Trials
Several pivotal clinical trials have established tamoxifen's efficacy:
- ATLAS Trial : This trial demonstrated that extending tamoxifen treatment from 5 to 10 years significantly reduces breast cancer recurrence and mortality rates .
- SOFT and TEXT Trials : These studies compared aromatase inhibitors with tamoxifen in premenopausal women, revealing lower recurrence rates with aromatase inhibitors but highlighting tamoxifen's continued relevance .
Side Effects and Considerations
While tamoxifen is effective, it is associated with several side effects:
Mechanism of Action
(E/Z)-Tamoxifen exerts its effects by competitively binding to estrogen receptors (ERα and ERβ), preventing estrogen from activating these receptors. This binding induces a conformational change in the receptor, altering the expression of estrogen-dependent genes and inhibiting cell proliferation. Additionally, (Z)-Tamoxifen can bind to DNA after metabolic activation, contributing to its anti-cancer effects .
Comparison with Similar Compounds
Comparison with Structural Analogs and Derivatives
Ferrocene-Linked Tamoxifen Derivatives
Modifications to Tamoxifen’s pharmacophore aim to enhance efficacy and reduce resistance. Ferrocene-linked derivatives, such as those in Table 1, exhibit improved redox properties and cytotoxicity. For example:
These derivatives show promise in preclinical models, particularly for ER-negative cancers and non-oncological applications (e.g., Parkinson’s disease) .
1,3-Diphenylpropan-1-one Derivatives
Compounds with structural similarity to Tamoxifen, such as 4a and 4h, demonstrate comparable cytotoxicity in MCF-7 cells but reduced toxicity in normal fibroblasts. Morpholine side chains improve selectivity over hydrophobic piperidinyl groups, suggesting safer profiles .
Comparison with Selective Estrogen Receptor Degraders (SERDs)
AZD9496 vs. Tamoxifen
AZD9496, an oral SERD, directly degrades ERα and circumvents resistance mechanisms (e.g., ESR1 mutations). In endocrine-resistant models, AZD9496 and fulvestrant show superior growth inhibition compared to Tamoxifen. Key advantages include:
- Efficacy: AZD9496 achieves 90% ER degradation in xenografts, surpassing Tamoxifen’s antagonism .
- Resistance Mitigation : Effective in Tamoxifen-resistant and fulvestrant-resistant models .
Fulvestrant vs. Tamoxifen
Fulvestrant, an intramuscular SERD, lacks Tamoxifen’s partial agonist effects. While both drugs inhibit ER+ tumor growth, fulvestrant’s irreversible ER degradation offers longer-term suppression but requires invasive administration .
Comparison with Aromatase Inhibitors (AIs)
Exemestane vs. Tamoxifen
Exemestane, a steroidal AI, suppresses estrogen synthesis in postmenopausal women. In the TEAM and IES trials, switching to exemestane after 2–3 years of Tamoxifen reduced recurrence by 32% compared to Tamoxifen alone . Advantages include:
- Mechanism : Targets estrogen production rather than ER signaling.
- Resistance Profile : Effective in Tamoxifen-resistant cases due to distinct action .
Key Research Findings and Clinical Implications
Biological Activity
Tamoxifen is a selective estrogen receptor modulator (SERM) widely used in the treatment and prevention of estrogen receptor-positive breast cancer. Its biological activity is primarily attributed to its ability to bind to estrogen receptors (ERs), leading to both anti-estrogenic and estrogen-like effects depending on the tissue context. This article delves into the biological activity of both the (E) and (Z) isomers of Tamoxifen, focusing on their metabolism, pharmacokinetics, and clinical implications.
Overview of Tamoxifen Isomers
Tamoxifen exists in two geometric isomers:
- (E)-Tamoxifen : The trans isomer, which is more commonly used in clinical settings.
- (Z)-Tamoxifen : The cis isomer, which has distinct pharmacological properties.
The differences in biological activity between these isomers can significantly influence their therapeutic efficacy and side effect profiles.
Metabolism and Pharmacokinetics
Tamoxifen undergoes extensive hepatic metabolism, primarily mediated by cytochrome P450 enzymes. Key metabolites include:
- 4-Hydroxytamoxifen : An active metabolite with anti-estrogenic properties.
- Endoxifen : Formed from 4-hydroxytamoxifen, it exhibits a higher affinity for ERs than Tamoxifen itself.
Table 1: Key Metabolites of Tamoxifen
Metabolite | Formation Enzymes | Biological Activity |
---|---|---|
Tamoxifen | N/A | SERM with mixed activity |
4-Hydroxytamoxifen | CYP2D6, CYP3A4 | Anti-estrogenic |
Endoxifen | CYP2D6, CYP3A4 | Strong anti-estrogenic |
Tamoxifen Bisphenol | UGT2B7, UGT1A8 | Induces CYP enzymes |
The formation of these metabolites can lead to varied pharmacological effects, influencing both efficacy and potential drug interactions. For instance, the induction of cytochrome P450 enzymes by certain metabolites may affect the metabolism of co-administered drugs, leading to altered therapeutic outcomes .
Estrogen Receptor Binding
Both (E)- and (Z)-Tamoxifen exhibit differential binding affinities to estrogen receptors. The (E)-isomer has a higher affinity for ERα compared to the (Z)-isomer. This difference plays a crucial role in their respective biological activities:
- (E)-Tamoxifen : Primarily functions as an antagonist in breast tissue but can act as an agonist in other tissues such as the uterus.
- (Z)-Tamoxifen : Exhibits weaker anti-estrogenic activity but may have unique effects that could be leveraged therapeutically.
Clinical Implications
Clinical studies have demonstrated that Tamoxifen significantly reduces the risk of breast cancer recurrence and mortality in women with ER-positive tumors. A pooled analysis from 20 trials indicated a substantial long-term benefit from Tamoxifen treatment, showing a one-third reduction in mortality over a 15-year follow-up period .
Case Studies
-
Long-Term Efficacy Study :
A study involving 12,894 patients showed that extending Tamoxifen treatment from 5 to 10 years reduced recurrence rates from 25% to 21.4% and mortality from 15% to 12.2% . -
Pharmacokinetic Interaction Study :
Research indicated that natural products like curcumin and EGCG could enhance the bioavailability of Tamoxifen by inhibiting first-pass metabolism, thus increasing its systemic exposure and potential efficacy against breast cancer .
Q & A
Basic Research Questions
Q. How do the structural differences between (E)- and (Z)-tamoxifen isomers influence experimental design in receptor-binding studies?
The (Z)-isomer of tamoxifen exhibits potent anti-estrogenic activity by binding to estrogen receptors (ERs), while the (E)-isomer is less active due to steric hindrance . To study isomer-specific effects, researchers should:
- Use high-performance liquid chromatography (HPLC) with chiral columns to isolate isomers .
- Validate binding affinity via competitive ERα/β assays, comparing IC50 values for each isomer .
- Incorporate molecular docking simulations to map spatial interactions between isomers and ER ligand-binding domains .
Q. What methodologies are recommended to quantify tamoxifen metabolites like (E/Z)-4-hydroxytamoxifen and endoxifen in preclinical models?
Key steps include:
- Sample preparation : Use liquid-liquid extraction with tert-butyl methyl ether to isolate metabolites from plasma/tissue homogenates .
- Analytical techniques : Employ LC-MS/MS with multiple reaction monitoring (MRM) for sensitive detection of 4-hydroxytamoxifen (LOQ: 0.1 ng/mL) and endoxifen (LOQ: 0.5 ng/mL) .
- CYP2D6 genotyping : Correlate metabolite levels with CYP2D6 activity in vitro using human liver microsomes or transfected cell lines .
Q. How should clinical trial protocols address interindividual variability in tamoxifen metabolism due to CYP2D6 polymorphisms?
- Stratify participants by CYP2D6 phenotype (e.g., poor, intermediate, extensive metabolizers) using PCR-based allele-specific amplification .
- Monitor steady-state plasma endoxifen concentrations (target: ≥ 5.9 ng/mL) to ensure therapeutic efficacy .
- Adjust adjuvant therapy duration (e.g., extending to 10 years for high-risk cohorts) based on longitudinal metabolite profiling .
Advanced Research Questions
Q. What experimental approaches resolve contradictions in studies linking endoxifen concentrations to tamoxifen efficacy?
Conflicting data arise from heterogeneous study designs and variable CYP2D6 activity . To address this:
- Conduct meta-analyses with standardized inclusion criteria (e.g., ER+ tumors, premenopausal status) .
- Use Mendelian randomization to isolate CYP2D6 genetic variants as instrumental variables .
- Validate findings in tamoxifen-resistant xenograft models, comparing endoxifen pharmacokinetics in CYP2D6-knockout vs. wild-type mice .
Q. How can transcriptomic data identify mechanisms of acquired tamoxifen resistance in ER+ breast cancer?
- Gene expression profiling : Apply cDNA microarrays or RNA-seq to compare tamoxifen-sensitive vs. resistant cell lines (e.g., MCF-7 vs. TAMR) .
- Pathway analysis : Use principal components analysis (PCA) to prioritize altered pathways (e.g., oxidative stress response via NQO1/GCLC upregulation) .
- Functional validation : Knock down outlier genes (e.g., HSF-1) using CRISPR/Cas9 and assess proliferation in estrogen-deprived media .
Q. What advanced techniques characterize tamoxifen-induced quinone methide adducts linked to DNA damage?
- Reactive metabolite trapping : Incubate tamoxifen with cytochrome P450 isoforms (e.g., CYP2B6) and glutathione ethyl ester (GSHEE) to stabilize quinone methides for LC-MS/MS detection .
- DNA adduct mapping : Use <sup>32</sup>P-postlabeling to identify covalent modifications in hepatic or endometrial tissues .
- Computational modeling : Simulate metabolic activation pathways using density functional theory (DFT) to predict electrophilic intermediates .
Q. Controversies and Emerging Directions
Q. Why do some studies question the clinical utility of endoxifen monitoring despite its high ER affinity?
Limitations include:
- Lack of consensus on therapeutic thresholds for endoxifen .
- Compensatory mechanisms in tamoxifen resistance (e.g., ER-independent signaling via NQO1) .
- Ethnic variability in CYP2D6 allele frequencies (e.g., *10/*41 variants in Asian populations) .
Recommendation : Combine pharmacogenomic testing with proteomic profiling of ERα phosphorylation status to refine predictive models .
Q. How can researchers mitigate confounding factors in long-term tamoxifen prophylaxis studies?
- Control for menopausal status : Stratify analyses by serum estradiol levels and ovarian function .
- Address off-target effects : Monitor endometrial cancer risk via transvaginal ultrasound and biopsy in high-dose cohorts .
- Cost-effectiveness re-evaluation : Use Markov models incorporating updated 20-year survival data from NSABP P-1 trial .
Properties
IUPAC Name |
2-[4-(1,2-diphenylbut-1-enyl)phenoxy]-N,N-dimethylethanamine | |
---|---|---|
Source | PubChem | |
URL | https://pubchem.ncbi.nlm.nih.gov | |
Description | Data deposited in or computed by PubChem | |
InChI |
InChI=1S/C26H29NO/c1-4-25(21-11-7-5-8-12-21)26(22-13-9-6-10-14-22)23-15-17-24(18-16-23)28-20-19-27(2)3/h5-18H,4,19-20H2,1-3H3 | |
Source | PubChem | |
URL | https://pubchem.ncbi.nlm.nih.gov | |
Description | Data deposited in or computed by PubChem | |
InChI Key |
NKANXQFJJICGDU-UHFFFAOYSA-N | |
Source | PubChem | |
URL | https://pubchem.ncbi.nlm.nih.gov | |
Description | Data deposited in or computed by PubChem | |
Canonical SMILES |
CCC(=C(C1=CC=CC=C1)C2=CC=C(C=C2)OCCN(C)C)C3=CC=CC=C3 | |
Source | PubChem | |
URL | https://pubchem.ncbi.nlm.nih.gov | |
Description | Data deposited in or computed by PubChem | |
Molecular Formula |
C26H29NO | |
Source | PubChem | |
URL | https://pubchem.ncbi.nlm.nih.gov | |
Description | Data deposited in or computed by PubChem | |
DSSTOX Substance ID |
DTXSID60860081 | |
Record name | 2-{4-[(1Z)-1,2-Diphenylbut-1-en-1-yl]phenoxy}-N,N-dimethylethan-1-amine | |
Source | EPA DSSTox | |
URL | https://comptox.epa.gov/dashboard/DTXSID60860081 | |
Description | DSSTox provides a high quality public chemistry resource for supporting improved predictive toxicology. | |
Molecular Weight |
371.5 g/mol | |
Source | PubChem | |
URL | https://pubchem.ncbi.nlm.nih.gov | |
Description | Data deposited in or computed by PubChem | |
CAS No. |
7728-73-6 | |
Record name | (E,Z)-Tamoxifen | |
Source | CAS Common Chemistry | |
URL | https://commonchemistry.cas.org/detail?cas_rn=7728-73-6 | |
Description | CAS Common Chemistry is an open community resource for accessing chemical information. Nearly 500,000 chemical substances from CAS REGISTRY cover areas of community interest, including common and frequently regulated chemicals, and those relevant to high school and undergraduate chemistry classes. This chemical information, curated by our expert scientists, is provided in alignment with our mission as a division of the American Chemical Society. | |
Explanation | The data from CAS Common Chemistry is provided under a CC-BY-NC 4.0 license, unless otherwise stated. | |
Retrosynthesis Analysis
AI-Powered Synthesis Planning: Our tool employs the Template_relevance Pistachio, Template_relevance Bkms_metabolic, Template_relevance Pistachio_ringbreaker, Template_relevance Reaxys, Template_relevance Reaxys_biocatalysis model, leveraging a vast database of chemical reactions to predict feasible synthetic routes.
One-Step Synthesis Focus: Specifically designed for one-step synthesis, it provides concise and direct routes for your target compounds, streamlining the synthesis process.
Accurate Predictions: Utilizing the extensive PISTACHIO, BKMS_METABOLIC, PISTACHIO_RINGBREAKER, REAXYS, REAXYS_BIOCATALYSIS database, our tool offers high-accuracy predictions, reflecting the latest in chemical research and data.
Strategy Settings
Precursor scoring | Relevance Heuristic |
---|---|
Min. plausibility | 0.01 |
Model | Template_relevance |
Template Set | Pistachio/Bkms_metabolic/Pistachio_ringbreaker/Reaxys/Reaxys_biocatalysis |
Top-N result to add to graph | 6 |
Feasible Synthetic Routes
Disclaimer and Information on In-Vitro Research Products
Please be aware that all articles and product information presented on BenchChem are intended solely for informational purposes. The products available for purchase on BenchChem are specifically designed for in-vitro studies, which are conducted outside of living organisms. In-vitro studies, derived from the Latin term "in glass," involve experiments performed in controlled laboratory settings using cells or tissues. It is important to note that these products are not categorized as medicines or drugs, and they have not received approval from the FDA for the prevention, treatment, or cure of any medical condition, ailment, or disease. We must emphasize that any form of bodily introduction of these products into humans or animals is strictly prohibited by law. It is essential to adhere to these guidelines to ensure compliance with legal and ethical standards in research and experimentation.