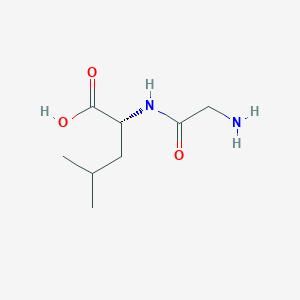
Glycyl-d-leucine
Overview
Description
Glycyl-d-leucine is a dipeptide composed of glycine and d-leucine joined by a peptide linkage This compound is a derivative of leucine, an essential amino acid, and glycine, the simplest amino acid
Preparation Methods
Synthetic Routes and Reaction Conditions
The synthesis of glycyl-d-leucine typically involves the formation of a peptide bond between glycine and d-leucine. One common method is the use of dicyclohexylcarbodiimide (DCC) as a coupling agent. The reaction is carried out in an organic solvent such as dimethylformamide (DMF) at room temperature. The carboxyl group of glycine is activated by DCC, allowing it to react with the amino group of d-leucine to form the dipeptide .
Industrial Production Methods
Industrial production of this compound can be achieved through fermentation processes using genetically engineered microorganisms. These microorganisms are designed to overproduce the desired dipeptide by optimizing the metabolic pathways involved in amino acid synthesis .
Chemical Reactions Analysis
Types of Reactions
Glycyl-d-leucine can undergo various chemical reactions, including:
Hydrolysis: The peptide bond can be hydrolyzed by proteolytic enzymes, resulting in the release of glycine and d-leucine.
Oxidation and Reduction: The compound can participate in redox reactions, although these are less common for dipeptides.
Substitution: The amino and carboxyl groups can be modified through substitution reactions to form derivatives with different properties.
Common Reagents and Conditions
Hydrolysis: Enzymatic hydrolysis using proteases such as trypsin or pepsin.
Oxidation: Mild oxidizing agents like hydrogen peroxide can be used.
Substitution: Reagents like acyl chlorides or anhydrides can be used for acylation reactions.
Major Products Formed
Hydrolysis: Glycine and d-leucine.
Oxidation: Oxidized derivatives of the amino acids.
Substitution: Acylated or other substituted derivatives of this compound.
Scientific Research Applications
Glycyl-d-leucine has several applications in scientific research:
Chemistry: Used as a model compound to study peptide bond formation and hydrolysis.
Biology: Investigated for its role in protein synthesis and degradation.
Medicine: Potential therapeutic applications due to its involvement in metabolic pathways.
Industry: Used in the production of peptide-based drugs and as a nutritional supplement
Mechanism of Action
The mechanism of action of glycyl-d-leucine involves its interaction with enzymes and receptors in the body. It can act as a substrate for proteolytic enzymes, leading to the release of its constituent amino acids. These amino acids can then participate in various metabolic pathways, including protein synthesis and energy production .
Comparison with Similar Compounds
Similar Compounds
Glycyl-l-leucine: Similar structure but contains l-leucine instead of d-leucine.
Glycyl-glycine: A simpler dipeptide composed of two glycine molecules.
Leucyl-glycine: The reverse sequence of glycyl-leucine
Uniqueness
Glycyl-d-leucine is unique due to the presence of d-leucine, which is less common in nature compared to l-leucine.
Biological Activity
Glycyl-d-leucine is a dipeptide composed of glycine and the d-enantiomer of leucine. It has garnered attention in biochemical research due to its potential biological activities, particularly in metabolic processes and as a modulator in various physiological functions. This article delves into the biological activity of this compound, supported by empirical data, case studies, and research findings.
- Molecular Formula : C₈H₁₆N₂O₃
- Molecular Weight : 172.22 g/mol
- CAS Number : 1715077
- Structure : this compound is characterized by the presence of a glycine moiety linked to d-leucine, which influences its biological interactions.
This compound exhibits various biological activities that can be attributed to its structural components:
- Amino Acid Transport : Similar to other amino acids, this compound is believed to interact with specific transporters in cell membranes, facilitating its uptake into cells. The transport mechanisms are crucial for its bioavailability and subsequent biological effects.
- Metabolic Impact : Research indicates that dipeptides like this compound can influence metabolic pathways through their hydrolysis into constituent amino acids, which participate in protein synthesis and energy metabolism.
- Regulatory Functions : Dipeptides may modulate signaling pathways related to growth and metabolism, notably through interactions with the mTORC1 pathway, which plays a pivotal role in cellular growth and proliferation.
In Vitro Studies
A study on the metabolism of glycyl-L-leucine in Escherichia coli demonstrated that the uptake of this compound leads to its rapid hydrolysis in young bacterial cultures but shows slower hydrolysis rates in aged cultures. This suggests that the biological activity may vary significantly depending on the physiological state of the organism (Meisler & Simmonds, 1963) .
Case Studies
- Muscle Growth and Recovery : In animal models, supplementation with leucine-rich dipeptides has been shown to enhance muscle protein synthesis and recovery post-exercise. This effect is likely mediated by increased activation of mTOR signaling pathways, which are critical for muscle hypertrophy (Duan et al., 2016) .
- Metabolic Disorders : Research indicates that leucine supplementation can improve insulin sensitivity and lipid metabolism, suggesting potential therapeutic applications for conditions such as type 2 diabetes and obesity (Duan et al., 2023) .
Comparative Biological Activity Table
Compound | Biological Activity | Mechanism of Action |
---|---|---|
This compound | Modulates amino acid transport | Interacts with membrane transporters |
Glycyl-L-leucine | Promotes muscle protein synthesis | Activates mTORC1 signaling pathway |
Leucine | Enhances metabolic health | Stimulates protein synthesis via mTORC1 |
Potential Side Effects
While this compound and related compounds show promise in various therapeutic applications, potential side effects must be considered. Increased intake of amino acids can lead to disturbances in amino acid balance within tissues, potentially causing metabolic dysregulation (PMC, 2022) . Long-term studies are necessary to fully understand the implications of dipeptide supplementation.
Properties
IUPAC Name |
(2R)-2-[(2-aminoacetyl)amino]-4-methylpentanoic acid | |
---|---|---|
Source | PubChem | |
URL | https://pubchem.ncbi.nlm.nih.gov | |
Description | Data deposited in or computed by PubChem | |
InChI |
InChI=1S/C8H16N2O3/c1-5(2)3-6(8(12)13)10-7(11)4-9/h5-6H,3-4,9H2,1-2H3,(H,10,11)(H,12,13)/t6-/m1/s1 | |
Source | PubChem | |
URL | https://pubchem.ncbi.nlm.nih.gov | |
Description | Data deposited in or computed by PubChem | |
InChI Key |
DKEXFJVMVGETOO-ZCFIWIBFSA-N | |
Source | PubChem | |
URL | https://pubchem.ncbi.nlm.nih.gov | |
Description | Data deposited in or computed by PubChem | |
Canonical SMILES |
CC(C)CC(C(=O)O)NC(=O)CN | |
Source | PubChem | |
URL | https://pubchem.ncbi.nlm.nih.gov | |
Description | Data deposited in or computed by PubChem | |
Isomeric SMILES |
CC(C)C[C@H](C(=O)O)NC(=O)CN | |
Source | PubChem | |
URL | https://pubchem.ncbi.nlm.nih.gov | |
Description | Data deposited in or computed by PubChem | |
Molecular Formula |
C8H16N2O3 | |
Source | PubChem | |
URL | https://pubchem.ncbi.nlm.nih.gov | |
Description | Data deposited in or computed by PubChem | |
DSSTOX Substance ID |
DTXSID001017042 | |
Record name | Glycyl-D-leucine | |
Source | EPA DSSTox | |
URL | https://comptox.epa.gov/dashboard/DTXSID001017042 | |
Description | DSSTox provides a high quality public chemistry resource for supporting improved predictive toxicology. | |
Molecular Weight |
188.22 g/mol | |
Source | PubChem | |
URL | https://pubchem.ncbi.nlm.nih.gov | |
Description | Data deposited in or computed by PubChem | |
CAS No. |
688-13-1 | |
Record name | Glycyl-D-leucine | |
Source | EPA DSSTox | |
URL | https://comptox.epa.gov/dashboard/DTXSID001017042 | |
Description | DSSTox provides a high quality public chemistry resource for supporting improved predictive toxicology. | |
Retrosynthesis Analysis
AI-Powered Synthesis Planning: Our tool employs the Template_relevance Pistachio, Template_relevance Bkms_metabolic, Template_relevance Pistachio_ringbreaker, Template_relevance Reaxys, Template_relevance Reaxys_biocatalysis model, leveraging a vast database of chemical reactions to predict feasible synthetic routes.
One-Step Synthesis Focus: Specifically designed for one-step synthesis, it provides concise and direct routes for your target compounds, streamlining the synthesis process.
Accurate Predictions: Utilizing the extensive PISTACHIO, BKMS_METABOLIC, PISTACHIO_RINGBREAKER, REAXYS, REAXYS_BIOCATALYSIS database, our tool offers high-accuracy predictions, reflecting the latest in chemical research and data.
Strategy Settings
Precursor scoring | Relevance Heuristic |
---|---|
Min. plausibility | 0.01 |
Model | Template_relevance |
Template Set | Pistachio/Bkms_metabolic/Pistachio_ringbreaker/Reaxys/Reaxys_biocatalysis |
Top-N result to add to graph | 6 |
Feasible Synthetic Routes
Q1: What is known about the solubility of Glycyl-d-leucine?
A1: Research has investigated the solubility of several cyclic dipeptides, including this compound, in water at 298.15 K []. This study found that this compound has a specific solubility at that temperature, which was then used to calculate thermodynamic properties like the molar Gibbs free energy of dissolution.
Q2: Can you explain more about how the solubility data was used to understand the thermodynamics of this compound dissolving in water?
A2: The researchers experimentally determined the solubility constant (Ksp) of this compound in water []. This constant reflects the equilibrium between the solid dipeptide and its dissolved form. From the Ksp, they were able to calculate the Gibbs free energy of dissolution (ΔG), which indicates the spontaneity of the dissolving process. They also utilized previously gathered data on enthalpy of dissolution (ΔH) and combined it with the new ΔG values to determine the entropy of dissolution (ΔS). These calculations offer insight into the energy changes and order/disorder shifts that happen when this compound dissolves in water.
Q3: Were any other dipeptides examined in this solubility study?
A3: Yes, the study looked at the solubilities of four other cyclic dipeptides: cyclic glycylglycine, cyclic d-alanylglycine, cyclic d-alanyl-d-alanine, and cyclic d-valyl-L-valine []. Comparing these results to this compound could provide insights into how structural variations among dipeptides influence their solubility behavior.
Disclaimer and Information on In-Vitro Research Products
Please be aware that all articles and product information presented on BenchChem are intended solely for informational purposes. The products available for purchase on BenchChem are specifically designed for in-vitro studies, which are conducted outside of living organisms. In-vitro studies, derived from the Latin term "in glass," involve experiments performed in controlled laboratory settings using cells or tissues. It is important to note that these products are not categorized as medicines or drugs, and they have not received approval from the FDA for the prevention, treatment, or cure of any medical condition, ailment, or disease. We must emphasize that any form of bodily introduction of these products into humans or animals is strictly prohibited by law. It is essential to adhere to these guidelines to ensure compliance with legal and ethical standards in research and experimentation.