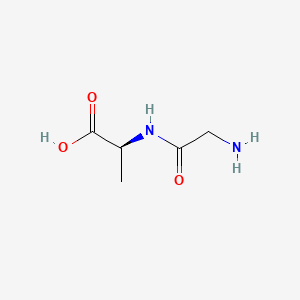
Glycyl-L-alanine
Overview
Description
Glycyl-L-alanine is a dipeptide composed of glycine and L-alanine. It is a simple yet significant compound in the field of biochemistry and molecular biology. Dipeptides like this compound are formed by the condensation of two amino acids, resulting in a peptide bond. This compound is often used in research to study peptide behavior, protein structure, and enzyme interactions.
Mechanism of Action
Target of Action
Glycyl-L-alanine is a dipeptide composed of glycine and L-alanine . It has been studied for its interaction with hydroxopentaaquarhodium(iii) ion .
Mode of Action
The interaction of this compound with its targets has been studied spectrophotometrically in aqueous medium . The reaction proceeds via two parallel paths, both of which are ligand dependent . The rate constants for these processes indicate an associative mode of activation for both the aqua ligand substitution processes .
Biochemical Pathways
It’s known that dipeptides like this compound can play roles in various biological processes . For instance, they can influence the structure of water around them, which may have implications for protein folding and stability .
Result of Action
It’s known that dipeptides can have various effects at the molecular and cellular levels, depending on their specific targets and modes of action .
Action Environment
The action of this compound, like that of other peptides, can be influenced by various environmental factors. For instance, the pH and temperature of the environment can affect the interaction of this compound with its targets . Furthermore, the presence of other molecules in the environment can also influence its action .
Biochemical Analysis
Biochemical Properties
Glycyl-L-alanine participates in biochemical reactions as a substrate. It interacts with various enzymes and proteins. For instance, it is involved in the reaction catalyzed by the enzyme dipeptidase, which hydrolyzes this compound into its constituent amino acids, glycine and L-alanine .
Cellular Effects
The effects of this compound on cells are primarily related to its role as a building block for proteins. As a dipeptide, it can be incorporated into larger polypeptides during protein synthesis, influencing cell function
Molecular Mechanism
At the molecular level, this compound exerts its effects through its participation in protein synthesis. It can bind to transfer RNA (tRNA) molecules during translation, facilitating the incorporation of its constituent amino acids into growing polypeptide chains .
Temporal Effects in Laboratory Settings
In laboratory settings, the effects of this compound can change over time, depending on factors such as concentration and environmental conditions. For instance, its solubility in water can vary with temperature
Metabolic Pathways
This compound is involved in the metabolic pathway of protein biosynthesis, where it can be incorporated into larger polypeptides. It can also be hydrolyzed into its constituent amino acids, glycine and L-alanine, which participate in various metabolic pathways .
Transport and Distribution
This compound can be transported across cell membranes by specific transporters, such as peptide transporters
Subcellular Localization
The subcellular localization of this compound is likely to be influenced by its role in protein synthesis. It can be found in the cytoplasm, where protein synthesis occurs
Preparation Methods
Synthetic Routes and Reaction Conditions
The synthesis of glycyl-L-alanine typically involves the coupling of glycine and L-alanine. One common method is the use of carbamate protecting groups. The process begins with the protection of the amino group of glycine using a carbamate such as t-butyloxycarbonyl (Boc). The protected glycine is then coupled with L-alanine using a coupling agent like N,N’-dicyclohexylcarbodiimide (DCC) to form the dipeptide bond .
Industrial Production Methods
Industrial production of this compound can be achieved through both chemical synthesis and biotechnological methods. Chemical synthesis involves the stepwise addition of amino acids, while biotechnological methods may use engineered microorganisms to produce the dipeptide. For example, Escherichia coli can be metabolically engineered to produce dipeptides by overexpressing specific enzymes and inactivating peptidases .
Chemical Reactions Analysis
Types of Reactions
Glycyl-L-alanine can undergo various chemical reactions, including:
Hydrolysis: The peptide bond can be hydrolyzed to yield the constituent amino acids, glycine and L-alanine.
Oxidation and Reduction: While the dipeptide itself is relatively stable, the amino acids can undergo oxidation and reduction reactions.
Substitution: The amino and carboxyl groups can participate in substitution reactions to form derivatives.
Common Reagents and Conditions
Hydrolysis: Acidic or basic conditions can be used to hydrolyze the peptide bond.
Oxidation: Oxidizing agents like hydrogen peroxide can be used.
Reduction: Reducing agents such as sodium borohydride may be employed.
Major Products
The major products of these reactions are typically the free amino acids, glycine and L-alanine, or their derivatives depending on the specific reaction conditions .
Scientific Research Applications
Glycyl-L-alanine has a wide range of applications in scientific research:
Chemistry: Used as a model compound to study peptide synthesis and behavior.
Biology: Helps in understanding protein folding and enzyme-substrate interactions.
Medicine: Investigated for its potential therapeutic properties and as a component in drug delivery systems.
Industry: Utilized in the production of peptide-based materials and as a standard in analytical techniques
Comparison with Similar Compounds
Similar Compounds
Glycylglycine: Another simple dipeptide composed of two glycine molecules.
L-alanyl-glycine: An isomer of glycyl-L-alanine with the same amino acids in reverse order.
L-alanyl-L-alanine: A dipeptide composed of two L-alanine molecules.
Uniqueness
This compound is unique due to its specific sequence of glycine and L-alanine, which influences its solubility, stability, and interaction with enzymes. The differences in melting enthalpy and solubility profiles between this compound and its isomers highlight its distinct properties .
Properties
IUPAC Name |
(2S)-2-[(2-aminoacetyl)amino]propanoic acid | |
---|---|---|
Source | PubChem | |
URL | https://pubchem.ncbi.nlm.nih.gov | |
Description | Data deposited in or computed by PubChem | |
InChI |
InChI=1S/C5H10N2O3/c1-3(5(9)10)7-4(8)2-6/h3H,2,6H2,1H3,(H,7,8)(H,9,10)/t3-/m0/s1 | |
Source | PubChem | |
URL | https://pubchem.ncbi.nlm.nih.gov | |
Description | Data deposited in or computed by PubChem | |
InChI Key |
VPZXBVLAVMBEQI-VKHMYHEASA-N | |
Source | PubChem | |
URL | https://pubchem.ncbi.nlm.nih.gov | |
Description | Data deposited in or computed by PubChem | |
Canonical SMILES |
CC(C(=O)O)NC(=O)CN | |
Source | PubChem | |
URL | https://pubchem.ncbi.nlm.nih.gov | |
Description | Data deposited in or computed by PubChem | |
Isomeric SMILES |
C[C@@H](C(=O)O)NC(=O)CN | |
Source | PubChem | |
URL | https://pubchem.ncbi.nlm.nih.gov | |
Description | Data deposited in or computed by PubChem | |
Molecular Formula |
C5H10N2O3 | |
Source | PubChem | |
URL | https://pubchem.ncbi.nlm.nih.gov | |
Description | Data deposited in or computed by PubChem | |
Related CAS |
25765-56-4 | |
Record name | L-Alanine, N-glycyl-, homopolymer | |
Source | CAS Common Chemistry | |
URL | https://commonchemistry.cas.org/detail?cas_rn=25765-56-4 | |
Description | CAS Common Chemistry is an open community resource for accessing chemical information. Nearly 500,000 chemical substances from CAS REGISTRY cover areas of community interest, including common and frequently regulated chemicals, and those relevant to high school and undergraduate chemistry classes. This chemical information, curated by our expert scientists, is provided in alignment with our mission as a division of the American Chemical Society. | |
Explanation | The data from CAS Common Chemistry is provided under a CC-BY-NC 4.0 license, unless otherwise stated. | |
DSSTOX Substance ID |
DTXSID501316691 | |
Record name | Glycyl-L-alanine | |
Source | EPA DSSTox | |
URL | https://comptox.epa.gov/dashboard/DTXSID501316691 | |
Description | DSSTox provides a high quality public chemistry resource for supporting improved predictive toxicology. | |
Molecular Weight |
146.14 g/mol | |
Source | PubChem | |
URL | https://pubchem.ncbi.nlm.nih.gov | |
Description | Data deposited in or computed by PubChem | |
CAS No. |
3695-73-6 | |
Record name | Glycyl-L-alanine | |
Source | CAS Common Chemistry | |
URL | https://commonchemistry.cas.org/detail?cas_rn=3695-73-6 | |
Description | CAS Common Chemistry is an open community resource for accessing chemical information. Nearly 500,000 chemical substances from CAS REGISTRY cover areas of community interest, including common and frequently regulated chemicals, and those relevant to high school and undergraduate chemistry classes. This chemical information, curated by our expert scientists, is provided in alignment with our mission as a division of the American Chemical Society. | |
Explanation | The data from CAS Common Chemistry is provided under a CC-BY-NC 4.0 license, unless otherwise stated. | |
Record name | Glycylalanine, L- | |
Source | ChemIDplus | |
URL | https://pubchem.ncbi.nlm.nih.gov/substance/?source=chemidplus&sourceid=0003695736 | |
Description | ChemIDplus is a free, web search system that provides access to the structure and nomenclature authority files used for the identification of chemical substances cited in National Library of Medicine (NLM) databases, including the TOXNET system. | |
Record name | Glycyl-L-alanine | |
Source | EPA DSSTox | |
URL | https://comptox.epa.gov/dashboard/DTXSID501316691 | |
Description | DSSTox provides a high quality public chemistry resource for supporting improved predictive toxicology. | |
Record name | N-glycylalanine | |
Source | European Chemicals Agency (ECHA) | |
URL | https://echa.europa.eu/substance-information/-/substanceinfo/100.020.927 | |
Description | The European Chemicals Agency (ECHA) is an agency of the European Union which is the driving force among regulatory authorities in implementing the EU's groundbreaking chemicals legislation for the benefit of human health and the environment as well as for innovation and competitiveness. | |
Explanation | Use of the information, documents and data from the ECHA website is subject to the terms and conditions of this Legal Notice, and subject to other binding limitations provided for under applicable law, the information, documents and data made available on the ECHA website may be reproduced, distributed and/or used, totally or in part, for non-commercial purposes provided that ECHA is acknowledged as the source: "Source: European Chemicals Agency, http://echa.europa.eu/". Such acknowledgement must be included in each copy of the material. ECHA permits and encourages organisations and individuals to create links to the ECHA website under the following cumulative conditions: Links can only be made to webpages that provide a link to the Legal Notice page. | |
Record name | GLYCYLALANINE, L- | |
Source | FDA Global Substance Registration System (GSRS) | |
URL | https://gsrs.ncats.nih.gov/ginas/app/beta/substances/2M7GF488BL | |
Description | The FDA Global Substance Registration System (GSRS) enables the efficient and accurate exchange of information on what substances are in regulated products. Instead of relying on names, which vary across regulatory domains, countries, and regions, the GSRS knowledge base makes it possible for substances to be defined by standardized, scientific descriptions. | |
Explanation | Unless otherwise noted, the contents of the FDA website (www.fda.gov), both text and graphics, are not copyrighted. They are in the public domain and may be republished, reprinted and otherwise used freely by anyone without the need to obtain permission from FDA. Credit to the U.S. Food and Drug Administration as the source is appreciated but not required. | |
Retrosynthesis Analysis
AI-Powered Synthesis Planning: Our tool employs the Template_relevance Pistachio, Template_relevance Bkms_metabolic, Template_relevance Pistachio_ringbreaker, Template_relevance Reaxys, Template_relevance Reaxys_biocatalysis model, leveraging a vast database of chemical reactions to predict feasible synthetic routes.
One-Step Synthesis Focus: Specifically designed for one-step synthesis, it provides concise and direct routes for your target compounds, streamlining the synthesis process.
Accurate Predictions: Utilizing the extensive PISTACHIO, BKMS_METABOLIC, PISTACHIO_RINGBREAKER, REAXYS, REAXYS_BIOCATALYSIS database, our tool offers high-accuracy predictions, reflecting the latest in chemical research and data.
Strategy Settings
Precursor scoring | Relevance Heuristic |
---|---|
Min. plausibility | 0.01 |
Model | Template_relevance |
Template Set | Pistachio/Bkms_metabolic/Pistachio_ringbreaker/Reaxys/Reaxys_biocatalysis |
Top-N result to add to graph | 6 |
Feasible Synthetic Routes
Q1: What is the molecular formula and weight of Glycyl-L-alanine?
A1: The molecular formula of this compound is C5H10N2O3, and its molecular weight is 146.15 g/mol.
Q2: How has computational chemistry been employed to study this compound?
A: Computational studies have played a vital role in understanding this compound's properties. For instance, ab initio quantum chemical calculations have been used to determine the low-energy conformers of this compound in solution and predict their NMR chemical shifts. [] This approach helps bridge the gap between experimental observations and theoretical understanding of the dipeptide's behavior in various environments. Additionally, the IPolQ scheme, a fixed point-charge approach, has proven effective in simulating the aggregation behavior of this compound in aqueous solution, offering insights into solute-solute and solute-solvent interactions. [, ]
Q3: What is the significance of studying the electrostatic potential and polarizability of this compound?
A: Understanding the electrostatic potential and polarizability of this compound is crucial for comprehending its interactions with other molecules. Studies utilizing functional-group electrostatic potentials (GEP) and distributed polarizabilities (GDP) have demonstrated a strong correlation between the dipeptide's structural features and its intermolecular interactions. [] This information is valuable for predicting the behavior of this compound in various chemical and biological environments.
Q4: Does this compound exhibit any unique material properties?
A: Yes, research has revealed intriguing material properties of this compound. Crystallization of this compound hydroiodide monohydrate in the polar point group 2 makes it capable of exhibiting pyroelectricity and second harmonic generation. [] This polymorph shows a pyroelectric coefficient as high as 45 µC/m2K at 345 K and a nonlinear optical effective coefficient of 0.14 pm/V. []
Q5: How does the thermal stability of this compound compare to its cyclic form?
A: Interestingly, while no longer in its cyclic form when crystallized in its polymorphic form, this compound hydroiodide monohydrate exhibits a thermal melting point (533 K) very close to that of its cyclic counterpart, cyclo-glycyl-L-alanine (531 K). [] This suggests a "thermal memory effect," where the dipeptide retains some structural characteristics from its initial cyclic form.
Q6: How does this compound interact with biological systems?
A: Studies have explored the interaction of this compound with various biological systems. For example, research has focused on its absorption in the human body, specifically comparing the absorption rates of this compound with its individual amino acid constituents (glycine and L-alanine) in the jejunum and ileum. [] Results indicate that while glycine and L-alanine are absorbed faster from the jejunum, this compound shows comparable absorption rates in both jejunum and ileum. [] This highlights the significance of oligopeptide transport mechanisms in the assimilation of protein breakdown products.
Q7: Are there any studies investigating the transport mechanisms of this compound in bacteria?
A: Yes, research has shown that this compound can be transported into Escherichia coli K12 cells via the oligopeptide transport system. This was confirmed by cross-resistance studies with tri-L-ornithine and competition experiments using other peptides known to utilize the same transport system. [] The study also revealed that modifying an inhibitor of glutamine synthetase, L-methionine-S-dioxide, into a tripeptide form by attaching it to this compound allows it to be transported via the oligopeptide system, potentially leading to a synergistic inhibitory effect. []
Q8: What role does this compound play in studying the antifungal activity of polyoxin A?
A: this compound has been instrumental in understanding the antifungal mechanism of polyoxin A. Studies have shown that while this compound can significantly reduce the uptake of polyoxin A by Alternaria kikuchiana, it does not interfere with the antibiotic's inhibitory effect on isolated chitin synthetase. [] This suggests that this compound's antagonistic effect is primarily due to its interference with polyoxin A's transport into the fungal cells.
Q9: Has this compound been used in studying enzyme kinetics and mechanisms?
A: Yes, this compound has been employed as a substrate to investigate the kinetic and mechanistic properties of enzymes. One study utilized this compound in conjunction with hydroxopentaaquarhodium(III) ions to explore the kinetics of their interaction. [] The research revealed a two-path mechanism for the reaction, with both paths being ligand-dependent, providing valuable insights into the enzyme's catalytic behavior.
Disclaimer and Information on In-Vitro Research Products
Please be aware that all articles and product information presented on BenchChem are intended solely for informational purposes. The products available for purchase on BenchChem are specifically designed for in-vitro studies, which are conducted outside of living organisms. In-vitro studies, derived from the Latin term "in glass," involve experiments performed in controlled laboratory settings using cells or tissues. It is important to note that these products are not categorized as medicines or drugs, and they have not received approval from the FDA for the prevention, treatment, or cure of any medical condition, ailment, or disease. We must emphasize that any form of bodily introduction of these products into humans or animals is strictly prohibited by law. It is essential to adhere to these guidelines to ensure compliance with legal and ethical standards in research and experimentation.