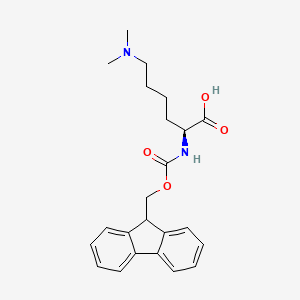
Fmoc-Lys(Me2)-OH
- Click on QUICK INQUIRY to receive a quote from our team of experts.
- With the quality product at a COMPETITIVE price, you can focus more on your research.
Overview
Description
Fmoc-Lys(Me2)-OH, also known as N-(9-fluorenylmethoxycarbonyl)-N,N-dimethyl-L-lysine, is a derivative of lysine, an essential amino acid. This compound is widely used in peptide synthesis, particularly in the solid-phase peptide synthesis (SPPS) method. The Fmoc group serves as a protecting group for the amino group, allowing for the stepwise construction of peptides.
Preparation Methods
Synthetic Routes and Reaction Conditions
The synthesis of Fmoc-Lys(Me2)-OH typically involves the protection of the lysine amino group with the Fmoc group. This is achieved through a reaction with 9-fluorenylmethoxycarbonyl chloride in the presence of a base such as diisopropylethylamine (DIPEA). The dimethylation of the lysine side chain is carried out using formaldehyde and a reducing agent like sodium cyanoborohydride .
Industrial Production Methods
In industrial settings, the production of this compound follows similar synthetic routes but on a larger scale. Automated peptide synthesizers are often employed to streamline the process, ensuring high purity and yield. The use of solid-phase synthesis allows for efficient separation of intermediates and minimizes the need for extensive purification steps .
Chemical Reactions Analysis
Types of Reactions
Fmoc-Lys(Me2)-OH undergoes various chemical reactions, including:
Deprotection: The Fmoc group can be removed using piperidine, revealing the free amino group for further peptide coupling.
Coupling Reactions: The free amino group can react with activated carboxyl groups of other amino acids to form peptide bonds.
Substitution Reactions: The dimethylated lysine side chain can participate in substitution reactions, although this is less common.
Common Reagents and Conditions
Major Products Formed
The primary products formed from these reactions are peptides with specific sequences, where this compound is incorporated at desired positions .
Scientific Research Applications
Chemistry
Fmoc-Lys(Me2)-OH is extensively used in the synthesis of complex peptides and proteins. Its incorporation into peptide sequences allows for the study of protein-protein interactions and the development of peptide-based drugs .
Biology
In biological research, this compound is used to create peptides that can act as enzyme substrates or inhibitors. These peptides are valuable tools for studying enzyme kinetics and mechanisms .
Medicine
The compound is used in the development of therapeutic peptides, which can target specific proteins involved in diseases. This has applications in cancer therapy, infectious diseases, and metabolic disorders .
Industry
This compound is used in the production of peptide-based materials, such as hydrogels, which have applications in drug delivery and tissue engineering .
Mechanism of Action
The mechanism of action of Fmoc-Lys(Me2)-OH in peptide synthesis involves the protection and deprotection of the amino group, allowing for the stepwise assembly of peptides. The Fmoc group protects the amino group during coupling reactions and is removed to reveal the free amino group for subsequent reactions . The dimethylated lysine side chain can influence the peptide’s conformation and interactions, affecting its biological activity .
Comparison with Similar Compounds
Similar Compounds
Fmoc-Lys(ivDde)-OH: This variant has an ivDde protecting group on the lysine side chain, allowing for orthogonal deprotection and selective modification.
Fmoc-Lys(5-Fam)-OH: This compound includes a fluorescent group, making it useful for studying peptide interactions and localization.
Uniqueness
Fmoc-Lys(Me2)-OH is unique due to its dimethylated lysine side chain, which can enhance the hydrophobicity and stability of peptides. This modification can influence the peptide’s biological activity and interactions, making it a valuable tool in peptide research and development .
Properties
Molecular Formula |
C23H28N2O4 |
---|---|
Molecular Weight |
396.5 g/mol |
IUPAC Name |
(2S)-6-(dimethylamino)-2-(9H-fluoren-9-ylmethoxycarbonylamino)hexanoic acid |
InChI |
InChI=1S/C23H28N2O4/c1-25(2)14-8-7-13-21(22(26)27)24-23(28)29-15-20-18-11-5-3-9-16(18)17-10-4-6-12-19(17)20/h3-6,9-12,20-21H,7-8,13-15H2,1-2H3,(H,24,28)(H,26,27)/t21-/m0/s1 |
InChI Key |
DCFIJZOUZZCQOO-NRFANRHFSA-N |
Isomeric SMILES |
CN(C)CCCC[C@@H](C(=O)O)NC(=O)OCC1C2=CC=CC=C2C3=CC=CC=C13 |
Canonical SMILES |
CN(C)CCCCC(C(=O)O)NC(=O)OCC1C2=CC=CC=C2C3=CC=CC=C13 |
Origin of Product |
United States |
Disclaimer and Information on In-Vitro Research Products
Please be aware that all articles and product information presented on BenchChem are intended solely for informational purposes. The products available for purchase on BenchChem are specifically designed for in-vitro studies, which are conducted outside of living organisms. In-vitro studies, derived from the Latin term "in glass," involve experiments performed in controlled laboratory settings using cells or tissues. It is important to note that these products are not categorized as medicines or drugs, and they have not received approval from the FDA for the prevention, treatment, or cure of any medical condition, ailment, or disease. We must emphasize that any form of bodily introduction of these products into humans or animals is strictly prohibited by law. It is essential to adhere to these guidelines to ensure compliance with legal and ethical standards in research and experimentation.