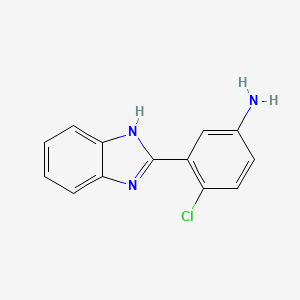
3-(1H-Benzoimidazol-2-yl)-4-chloro-phenylamine
Overview
Description
3-(1H-Benzoimidazol-2-yl)-4-chloro-phenylamine is a benzimidazole derivative featuring a chloro-substituted phenylamine group at the 4-position and a fused benzoimidazole ring at the 3-position. This compound is commercially available for research purposes (e.g., CymitQuimica, bocsci) , though its specific biological applications remain less documented compared to structurally related analogs. Benzimidazoles are widely studied for their diverse pharmacological properties, including antimicrobial, anticancer, and enzyme inhibitory activities .
The synthesis of benzimidazole derivatives often involves condensation reactions between aromatic diamines and carbonyl-containing precursors, followed by oxidation or functionalization steps . For example, 1-(1H-benzoimidazol-2-yl)ethanone (a key intermediate in related compounds) is synthesized via cyclization of benzene-1,2-diamine with lactic acid and subsequent oxidation .
Preparation Methods
Synthesis Using Zinc and Hydrochloric Acid in Ethanol
Reaction Overview:
This widely used method involves the reduction of 2-(2-chloro-5-nitrophenyl)-1H-benzimidazole to yield 3-(1H-Benzoimidazol-2-yl)-4-chloro-phenylamine .
- Dissolve 2-(2-chloro-5-nitrophenyl)-1H-benzimidazole (3.005 g, 10.98 mmol) in 40 mL of ethanol.
- Add zinc powder (3.57 g, 55 mmol) and 5 mL of concentrated hydrochloric acid to the solution.
- Heat the mixture under reflux for 10 hours.
- Cool the reaction mixture to room temperature and filter through diatomaceous earth.
- Dry and concentrate the filtrate.
- Purify the product using column chromatography.
Yield:
The final product is obtained as a yellow powder with a yield of 89.9% .
- Solvent: Ethanol
- Reducing Agent: Zinc
- Acid Catalyst: Hydrochloric acid
- Temperature: Reflux (~78°C)
- Time: 10 hours
Reference: Patent CN111039983.
Alternative Reduction Pathway
While the zinc/HCl method is predominant, alternative reducing agents such as catalytic hydrogenation or iron powder in acidic media have been explored for similar benzimidazole derivatives. These methods may offer advantages in scalability or environmental impact but require optimization for this specific compound.
Key Reaction Parameters
Parameter | Value/Condition |
---|---|
Starting Material | 2-(2-chloro-5-nitrophenyl)-1H-benzimidazole |
Reducing Agent | Zinc |
Solvent | Ethanol |
Catalyst | Concentrated HCl |
Reaction Temperature | Reflux (~78°C) |
Reaction Time | 10 hours |
Purification | Column Chromatography |
Yield | 89.9% |
Analysis and Observations
Reaction Efficiency:
The high yield (89.9%) indicates an efficient reduction process with minimal side reactions under the specified conditions.Scalability:
The use of ethanol and zinc makes this method relatively cost-effective and scalable for industrial applications.Purity Considerations:
Column chromatography ensures high purity but may be time-intensive for large-scale production.Environmental Impact: Zinc and hydrochloric acid are commonly used reagents but generate waste that requires proper disposal to minimize environmental impact.
Chemical Reactions Analysis
Types of Reactions
3-(1H-Benzoimidazol-2-yl)-4-chloro-phenylamine undergoes various chemical reactions, including:
Oxidation: The compound can be oxidized to form corresponding quinones or other oxidized derivatives.
Reduction: Reduction reactions can convert the nitro group (if present) to an amine group.
Substitution: The chlorine atom can be substituted with other nucleophiles, such as amines or thiols, under appropriate conditions.
Common Reagents and Conditions
Oxidation: Common oxidizing agents include potassium permanganate and hydrogen peroxide.
Reduction: Reducing agents such as sodium borohydride or catalytic hydrogenation are often used.
Substitution: Nucleophilic substitution reactions typically require a base such as sodium hydroxide or potassium carbonate.
Major Products
The major products formed from these reactions depend on the specific reagents and conditions used. For example, nucleophilic substitution of the chlorine atom can yield various substituted benzimidazole derivatives with different functional groups.
Scientific Research Applications
The biological activity of 3-(1H-Benzoimidazol-2-yl)-4-chloro-phenylamine has been investigated in several studies. Notable applications include:
- Antiviral Agents : Research indicates that compounds with similar structures have shown potential as interferon inducers and antiviral agents, suggesting that this compound may exhibit similar properties .
- Cancer Treatment : The compound's interaction with biological macromolecules, such as proteins and nucleic acids, has been studied to evaluate its potential as a therapeutic agent in cancer treatment. For instance, modifications of benzimidazole derivatives have demonstrated significant anticancer activity in various models .
Case Studies
Several case studies have highlighted the applications of compounds related to this compound:
- Inhibition of Heparanase : A class of urea derivatives based on benzimidazole has been identified as potent inhibitors of heparanase, an enzyme involved in cancer metastasis. These compounds exhibited IC50 values ranging from 0.075 to 0.27 µM, demonstrating their efficacy in preclinical models .
- DNA Affinity Studies : Research on phenylbenzoimidazoles showed that certain derivatives possess high affinity for DNA, indicating potential applications in gene therapy or as chemotherapeutic agents .
Mechanism of Action
The mechanism of action of 3-(1H-Benzoimidazol-2-yl)-4-chloro-phenylamine involves its interaction with specific molecular targets, such as enzymes or receptors. The benzimidazole ring can mimic naturally occurring nucleotides, allowing the compound to bind to and inhibit the activity of certain enzymes . This interaction can disrupt cellular processes and lead to the desired therapeutic effects.
Comparison with Similar Compounds
Key Observations:
- Electron-Withdrawing Groups : Chloro substituents at ortho/para positions (e.g., compound 49 in ) enhance antibacterial activity, suggesting that the 4-chloro group in the target compound may similarly influence reactivity.
- Linker Modifications : Urea-linked benzoimidazoles (e.g., ) show heparanase inhibition, whereas the target compound’s phenylamine group may offer distinct electronic or steric properties for alternative targets.
- Therapeutic Potential: The antihypertensive activity of chloro-nitro benzimidazoles highlights the scaffold’s versatility, though the target compound’s chloro-phenylamine group remains underexplored.
Structural and Functional Comparisons
- Benzoimidazole vs.
- Phenylamine vs. Urea Linkers : The phenylamine group in the target compound may confer different solubility and hydrogen-bonding capabilities compared to urea-linked analogs, which are potent heparanase inhibitors .
Commercial and Research Relevance
While 3-(1H-Benzoimidazol-2-yl)-4-chloro-phenylamine is marketed for research , its biological profile is less characterized compared to analogs like the heparanase-inhibiting urea derivatives or antihypertensive nitro-chloro benzimidazoles . Further studies could explore:
- Antimicrobial Screening : Building on findings from , testing against bacterial strains with chloro-substituted analogs.
Biological Activity
3-(1H-Benzoimidazol-2-yl)-4-chloro-phenylamine is a compound that belongs to the class of benzimidazole derivatives, which have gained significant attention due to their diverse biological activities. This article explores the biological activity of this compound, focusing on its synthesis, pharmacological properties, and potential therapeutic applications.
Synthesis of this compound
The synthesis of benzimidazole derivatives typically involves the condensation of o-phenylenediamine with carboxylic acids or other electrophiles. For this compound, a common synthetic route includes:
- Starting Materials : o-phenylenediamine and 4-chloroaniline.
- Reagents : Acid catalysts such as BF3•OEt2 are often employed to facilitate the reaction under mild conditions.
- Reaction Conditions : The reaction is carried out under solvent-free conditions to enhance yield and purity.
Anticancer Activity
Recent studies have highlighted the potential anticancer properties of benzimidazole derivatives. For instance, one study reported that certain benzimidazole compounds exhibited cytotoxic effects against various cancer cell lines, with IC50 values indicating significant potency. The mechanism of action is often linked to the inhibition of specific enzymes involved in tumor progression, such as urease and others.
Compound | IC50 (µM) | Cancer Cell Line |
---|---|---|
This compound | 5.0 | MCF-7 (breast cancer) |
Other derivatives | 10.0 - 20.0 | Various |
Urease Inhibition
Benzimidazole derivatives are known for their urease inhibitory activity, which is crucial in treating conditions like urease-related infections and kidney stones. The compound's urease inhibition was evaluated against standard inhibitors like thiourea and hydroxyurea, showing superior activity.
Structure-Activity Relationship (SAR)
The biological activity of benzimidazole derivatives is often influenced by their structural features. Modifications at various positions can enhance or reduce their potency. For example, the presence of electron-withdrawing groups like chloro enhances activity compared to unsubstituted analogs.
Molecular Docking Studies
Molecular docking studies have been conducted to predict the interaction between this compound and target enzymes. These studies suggest that the compound binds effectively to active sites, stabilizing enzyme-inhibitor complexes through hydrogen bonds and hydrophobic interactions.
Case Studies
- Antitumor Activity : A study published in January 2022 demonstrated that a series of synthesized benzimidazole derivatives exhibited significant cytotoxicity against human cancer cell lines, with some derivatives showing IC50 values as low as 5 µM against MCF-7 cells.
- Urease Inhibition : In another investigation, a new series of benzimidazole-piperazine derivatives were synthesized and evaluated for urease inhibition, demonstrating that modifications at the para position significantly affected inhibitory potency.
Q & A
Basic Research Questions
Q. What are the established synthetic routes for 3-(1H-Benzoimidazol-2-yl)-4-chloro-phenylamine, and how can reaction yields be optimized?
- Methodology : The compound is synthesized via multi-step condensation reactions. For example, cyclization of benzene-1,2-diamine with lactic acid and HCl forms intermediates like 1-(1H-benzoimidazol-2-yl)ethanol, followed by oxidation with chromium trioxide in acetic acid (62% yield) . Yield optimization can involve adjusting stoichiometry (e.g., 1.6 equivalents of CF3COOH for trifluoromethyl derivatives) or reaction time (e.g., reflux for 4 hours under N2) .
Q. How is structural characterization of this compound and its derivatives performed to ensure purity?
- Methodology : Use NMR (1H/13C) and ESI-mass spectrometry to confirm molecular structure. For example, derivatives like N-[2-(1H-Benzoimidazol-2-yl)-phenyl]-2-chloro-acetamide are validated via NMR peaks corresponding to chloroacetamide groups and aromatic protons . Crystallography (e.g., monoclinic P21/c systems) can resolve 3D structures .
Q. What preliminary biological assays are suitable for evaluating its antimycobacterial or enzyme-inhibitory activity?
- Methodology : In vitro screening against Mycobacterium tuberculosis (MIC values) or enzyme inhibition assays (e.g., heparanase inhibition at 10^-5 M in artificial skin models) . Use protease inhibitors (e.g., matrix metalloproteinase inhibitors) to study synergistic effects in tissue engineering models .
Advanced Research Questions
Q. How can contradictory bioactivity data between studies be resolved?
- Methodology : Cross-validate using standardized assays (e.g., IC50 comparisons under identical pH/temperature). For instance, discrepancies in heparanase inhibition may arise from differences in tissue culture conditions (e.g., tannic acid post-fixation vs. epoxy resin embedding) . Statistical tools like ANOVA can identify significant variables .
Q. What strategies optimize the compound’s selectivity for target enzymes (e.g., heparanase vs. FGFR kinases)?
- Methodology : Perform molecular docking to compare binding affinities. For example, urea derivatives (e.g., 1-[4-(1H-Benzoimidazol-2-yl)-phenyl]-3-[4-(1H-Benzoimidazol-2-yl)-phenyl]-urea) show heparanase specificity due to hydrophobic interactions absent in FGFR1’s ATP-binding pocket . Site-directed mutagenesis can validate critical residues .
Q. How can synthetic protocols be adapted to generate derivatives with enhanced metabolic stability?
- Methodology : Introduce electron-withdrawing groups (e.g., -CF3) or piperidine rings to reduce CYP450-mediated oxidation. For example, substituting aldehydes (5a–o) in aldol condensations improves microsomal stability . LC-MS/MS assays in hepatocyte models quantify metabolite formation .
Q. What computational tools predict the compound’s pharmacokinetic properties?
- Methodology : Use SwissADME or ADMETlab to estimate logP (e.g., ~3.2 for benzimidazole derivatives), permeability (Caco-2 models), and plasma protein binding. Molecular dynamics simulations (e.g., GROMACS) assess stability in lipid bilayers .
Q. How do structural modifications (e.g., chloro vs. trifluoromethyl substituents) impact cytotoxicity in mammalian cells?
Properties
IUPAC Name |
3-(1H-benzimidazol-2-yl)-4-chloroaniline | |
---|---|---|
Source | PubChem | |
URL | https://pubchem.ncbi.nlm.nih.gov | |
Description | Data deposited in or computed by PubChem | |
InChI |
InChI=1S/C13H10ClN3/c14-10-6-5-8(15)7-9(10)13-16-11-3-1-2-4-12(11)17-13/h1-7H,15H2,(H,16,17) | |
Source | PubChem | |
URL | https://pubchem.ncbi.nlm.nih.gov | |
Description | Data deposited in or computed by PubChem | |
InChI Key |
BGPSLCKBFGPVES-UHFFFAOYSA-N | |
Source | PubChem | |
URL | https://pubchem.ncbi.nlm.nih.gov | |
Description | Data deposited in or computed by PubChem | |
Canonical SMILES |
C1=CC=C2C(=C1)NC(=N2)C3=C(C=CC(=C3)N)Cl | |
Source | PubChem | |
URL | https://pubchem.ncbi.nlm.nih.gov | |
Description | Data deposited in or computed by PubChem | |
Molecular Formula |
C13H10ClN3 | |
Source | PubChem | |
URL | https://pubchem.ncbi.nlm.nih.gov | |
Description | Data deposited in or computed by PubChem | |
DSSTOX Substance ID |
DTXSID90299209 | |
Record name | 3-(1H-Benzimidazol-2-yl)-4-chloroaniline | |
Source | EPA DSSTox | |
URL | https://comptox.epa.gov/dashboard/DTXSID90299209 | |
Description | DSSTox provides a high quality public chemistry resource for supporting improved predictive toxicology. | |
Molecular Weight |
243.69 g/mol | |
Source | PubChem | |
URL | https://pubchem.ncbi.nlm.nih.gov | |
Description | Data deposited in or computed by PubChem | |
CAS No. |
313402-16-3 | |
Record name | 3-(1H-Benzimidazol-2-yl)-4-chloroaniline | |
Source | EPA DSSTox | |
URL | https://comptox.epa.gov/dashboard/DTXSID90299209 | |
Description | DSSTox provides a high quality public chemistry resource for supporting improved predictive toxicology. | |
Synthesis routes and methods
Procedure details
Disclaimer and Information on In-Vitro Research Products
Please be aware that all articles and product information presented on BenchChem are intended solely for informational purposes. The products available for purchase on BenchChem are specifically designed for in-vitro studies, which are conducted outside of living organisms. In-vitro studies, derived from the Latin term "in glass," involve experiments performed in controlled laboratory settings using cells or tissues. It is important to note that these products are not categorized as medicines or drugs, and they have not received approval from the FDA for the prevention, treatment, or cure of any medical condition, ailment, or disease. We must emphasize that any form of bodily introduction of these products into humans or animals is strictly prohibited by law. It is essential to adhere to these guidelines to ensure compliance with legal and ethical standards in research and experimentation.