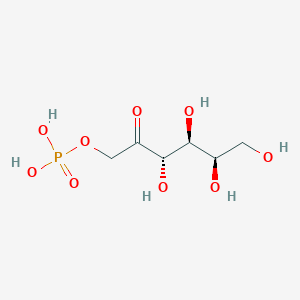
D-Tagatose 1-phosphate
- Click on QUICK INQUIRY to receive a quote from our team of experts.
- With the quality product at a COMPETITIVE price, you can focus more on your research.
Overview
Description
D-Tagatose 1-phosphate (Tag 1-P) is a phosphorylated derivative of the rare ketohexose D-tagatose, a C-4 epimer of D-fructose. It serves as a critical intermediate in bacterial carbohydrate catabolism, particularly in Gram-negative enterobacteria (e.g., Escherichia coli, Klebsiella oxytoca) and some Gram-positive bacteria like Bacillus licheniformis . Tag 1-P is generated via the phosphotransferase system (PTS), where D-tagatose is transported into the cell and phosphorylated at the C-1 position . Subsequent phosphorylation by tagatose 1-phosphate kinase (TagK) yields D-tagatose 1,6-bisphosphate (Tag 1,6-BP), which is cleaved by a class II aldolase (GatYZ) into dihydroxyacetone phosphate (DHAP) and D-glyceraldehyde 3-phosphate (G3P), feeding into glycolysis .
Preparation Methods
Synthetic Routes and Reaction Conditions: D-Tagatose 1-phosphate can be synthesized enzymatically using the phosphoenolpyruvate:sugar phosphotransferase system (PEP-PTS). In this system, the high-energy phosphoryl moiety of phosphoenolpyruvate is transferred sequentially to two general cytoplasmic proteins, Enzyme I and HPr, before being transferred to the sugar-specific Enzyme II, which phosphorylates D-tagatose to form this compound .
Industrial Production Methods: Industrial production of this compound typically involves the use of whole cells and isolated enzymes as catalysts under mild reaction conditions. This biocatalytic approach is preferred due to its high efficiency and environmental friendliness. The process often employs enzymes such as tagatose 4-epimerase, galactitol dehydrogenase, and L-arabinose isomerase to achieve the interconversion between monosaccharides and sugar alcohols .
Chemical Reactions Analysis
Metabolic Pathways and Degradation
Tag-1P enters glycolysis/gluconeogenesis via cleavage by aldolase B , though with lower efficiency compared to fructose-1-phosphate:
-
Cleavage products: Tag-1P is metabolized into dihydroxyacetone phosphate (DHAP) and glyceraldehyde, which feed into gluconeogenesis. Studies in rat hepatocytes show glucose production rates from Tag-1P are ~50% of those from fructose-1-phosphate .
-
ATP depletion: Prolonged accumulation of Tag-1P in hepatocytes reduces ATP levels by 30–40% and inorganic phosphate by 60%, indicating slower metabolic clearance compared to fructose derivatives .
Table 1: NMR Chemical Shifts of Tagatose 1-Phosphate (Tag-1P) vs. Related Compounds
Property | Tag-1P (pH 7.5, 25°C) | D-Tagatose (pH 6.5, -10°C) | Tagatose 6-Phosphate (pH 7.5) |
---|---|---|---|
1H Chemical Shift (ppm) | 3.63 (axial), 3.98 (eq) | 3.48 (axial), 3.70 (eq) | 4.11 (H6a), 4.03 (H6e) |
13C Chemical Shift (ppm) | 98.34 (C2) | 98.39 (C2) | 105.39 (C2) |
Dominant tautomer | α-pyranose (71%) | α-pyranose (72%) | β-pyranose (68%) |
Key observations:
-
Tag-1P exists primarily in the α-pyranose form in solution, distinct from tagatose 6-phosphate’s β-pyranose dominance .
-
The C2 carbon in Tag-1P shows a downfield 13C shift (98.34 ppm) compared to D-tagatose (98.39 ppm) .
Stability and Byproduct Formation
-
Thermal degradation: At neutral pH and 40°C, aqueous Tag-1P solutions lose <5% over six months but exhibit browning due to Maillard reaction participation .
-
Enzymatic byproducts: In Escherichia coli systems, competing pathways produce D-mannose (0.8 g/L) and D-psicose (1.1 g/L) alongside Tag-1P during bioproduction .
Health Implications
-
Uric acid elevation: Slow Tag-1P cleavage in hepatocytes increases purine degradation, raising serum uric acid levels—a mechanism analogous to hereditary fructose intolerance .
-
Phosphorus depletion: Human studies show transient serum inorganic phosphorus reduction after Tag-1P accumulation, linked to ATP sequestration .
These findings underscore Tag-1P’s dual role as a metabolic intermediate and modulator of cellular energy dynamics, with implications for both industrial biocatalysis and human carbohydrate metabolism disorders.
Scientific Research Applications
D-Tagatose-1-phosphate is an intermediate in the metabolism of D-tagatose, a low-glycemic sweetener with potential benefits for managing type 2 diabetes and obesity . While the molecular mechanisms are not fully understood , D-tagatose-1-phosphate plays a role in glycogen synthesis and glucose metabolism .
Effects on Glycogen Metabolism
D-Tagatose-1-phosphate influences glycogen metabolism by :
- Stimulating glucokinase: It promotes the activity of glucokinase, which results in increased phosphorylation of glucose to glucose-1-phosphate, activating glycogen synthase and mobilizing glucose to glycogen .
- Inhibiting glycogen phosphorylase: It inhibits glycogen phosphorylase activity, preventing glycogen breakdown .
- Slowing degradation: D-tagatose-1-phosphate is degraded slower than D-fructose-1-phosphate .
Impact on Blood Glucose
D-Tagatose has demonstrated potential in reducing blood glucose levels . Studies suggest the following :
- HbA1c Reduction: Clinical trials have shown statistically significant reductions in HbA1c levels in diabetic patients treated with D-tagatose compared to placebo .
- Postprandial Hyperglycemia: Supplementation with D-tagatose has shown to have a reducing effect on postprandial hyperglycemia in hyperglycemic individuals .
Safety and Efficacy Studies
Clinical trials have assessed the safety and efficacy of D-tagatose in managing type 2 diabetes :
- A phase III clinical trial demonstrated statistically significant reductions in HbA1c levels in patients with mild type 2 diabetes over a 10-month treatment period .
- D-tagatose was found to be effective in reducing HbA1c levels when administered at a dose of 15 g three times a day before meals for two months .
Potential Concerns
- Uric Acid Levels: Studies indicate that D-tagatose might increase plasma uric acid concentrations .
- Liver Effects: Animal studies have shown increased liver weights in rats given D-tagatose .
Production
- D-tagatose can be produced from lactose using engineered yeast strains . In this process, D-galactose is converted into D-tagatose .
Energy Conversion
Mechanism of Action
The mechanism of action of D-tagatose 1-phosphate involves its role as a substrate in the phosphotransferase system. It is phosphorylated by tagatose-1-phosphate kinase, which facilitates its conversion into other phosphorylated intermediates. These intermediates participate in various metabolic pathways, influencing processes such as glycolysis and glycogen synthesis . D-tagatose also affects sugar metabolism by inhibiting key enzymes, such as β-glucosidase and fructokinase, thereby impacting microbial growth and metabolism .
Comparison with Similar Compounds
Structural and Metabolic Pathway Comparisons
Table 1: Key Structural and Pathway Differences
Key Observations:
- Phosphorylation Position : Tag 1-P and Tag 6-P differ in phosphorylation sites, leading to distinct catabolic routes. Tag 1-P is phosphorylated to Tag 1,6-BP, while Tag 6-P is epimerized to fructose 6-phosphate .
- Enzymatic Specificity : Gram-negative bacteria utilize class II aldolases (GatYZ) for Tag 1,6-BP cleavage, whereas S. aureus employs class I aldolases for Tag 6-P processing .
- Prevalence : The Tag 1-P pathway dominates in Gram-negative bacteria (~60% prevalence), while the Tag 6-P pathway is niche (~20%) and restricted to select Gram-positive species .
Physicochemical Properties
Table 2: NMR Characterization of Phosphorylated Sugars
Key Observations:
- Tag 1-P exhibits distinct $^{31}$P chemical shifts and coupling constants compared to Tag 6-P, enabling differentiation via NMR .
- The C-1 phosphate group in Tag 1-P influences molecular conformation, impacting substrate specificity for kinases like TagK .
Biotechnological Implications
- Enzyme Engineering : Class II aldolases (GatYZ) from Tag 1-P pathways are thermostable and pH-tolerant, making them superior for industrial applications compared to class I enzymes .
- Metabolic Engineering : Redirecting Tag 1-P flux in B. licheniformis enhances D-tagatose production, leveraging its high pathway efficiency .
Biological Activity
D-Tagatose 1-phosphate (Tag-1P) is an important metabolite in the carbohydrate metabolism of certain organisms, particularly within the context of its role as a substrate in the phosphotransferase system (PTS). This article explores its biological activity, metabolic pathways, and relevant case studies that highlight its physiological effects.
Overview of this compound
D-Tagatose is a monosaccharide and a stereoisomer of D-fructose. Upon ingestion, it is phosphorylated to this compound, which plays a crucial role in various metabolic pathways. The phosphorylation occurs primarily in the liver through the action of fructokinase, leading to significant physiological effects due to its accumulation and subsequent metabolic fate.
Metabolic Pathways
The metabolism of D-tagatose involves several key steps:
- Phosphorylation : D-tagatose is converted into this compound by fructokinase.
- Glycolytic Intermediates : Tag-1P can be further processed into D-tagatose 1,6-bisphosphate (Tag 1,6-BP) by tagatose-1-phosphate kinase, which is then hydrolyzed by aldolase to yield glycolytic intermediates like glyceraldehyde 3-phosphate (G3P) and dihydroxyacetone phosphate (DHAP) .
Table 1: Key Metabolic Pathways Involving this compound
Step | Enzyme | Product |
---|---|---|
Phosphorylation | Fructokinase | This compound |
Conversion | Tagatose-1-phosphate kinase | D-tagatose 1,6-bisphosphate |
Hydrolysis | Aldolase | G3P and DHAP |
Liver Metabolism
Research indicates that moderate intake of D-tagatose influences liver metabolism significantly. A study using magnetic resonance spectroscopy showed that administration of D-tagatose led to a reduction in ATP levels and an increase in uric acid production due to phosphate trapping in the liver . This effect is particularly relevant for individuals with hereditary fructose intolerance, as it mimics similar metabolic disturbances.
Toxicological Studies
Toxicological assessments have shown that high doses of D-tagatose can lead to increased liver weights and hypertrophy in animal models, specifically Sprague-Dawley rats . However, these changes were reversible upon cessation of intake. Notably, Wistar rats exhibited less sensitivity to these effects, suggesting strain-specific responses to D-tagatose .
Clinical Trials
In clinical settings, D-tagatose has demonstrated potential benefits for glycemic control. In a randomized controlled trial involving type 2 diabetes patients, administration of D-tagatose resulted in significant reductions in HbA1c levels over time . The proposed mechanism involves the inhibition of hepatic glycogenolysis and promotion of glycogen synthesis through increased glucokinase activity mediated by Tag-1P.
Q & A
Basic Research Questions
Q. What experimental methods are used to synthesize and characterize D-tagatose 1-phosphate (DT1P) in vitro?
DT1P is synthesized enzymatically using kinases from pathways such as the Bacillus licheniformis phosphotransferase system. Key characterization techniques include:
- Nuclear Magnetic Resonance (NMR) : 1D 31P and 2D 1H-31P NMR to resolve phosphorylation patterns and confirm stereochemistry .
- Mass spectrometry : To verify molecular weight and purity.
- Enzymatic assays : Coupling reactions with glycerophosphate dehydrogenase or triose phosphate isomerase to validate functional activity .
Q. How is the structural configuration of DT1P distinguished from other phosphorylated sugars (e.g., fructose 1-phosphate)?
13C-31P coupling constants and chemical shift analysis in NMR differentiate DT1P from isomers. For example:
- DT1P exhibits distinct 31P shifts (~3–5 ppm) compared to fructose 1-phosphate (~5–7 ppm) due to differences in phosphate group orientation .
- Comparative 2D NMR with reference compounds (e.g., D-tagatose-6-phosphate) resolves anomeric carbon configurations .
Advanced Research Questions
Q. What metabolic pathways involve DT1P, and how do they differ across bacterial species?
DT1P is a substrate in the tagatose catabolic pathway of Bacillus licheniformis, where it is phosphorylated by Tagatose-1-phosphate kinase (TagK) . Contrastingly, Klebsiella pneumoniae metabolizes DT1P via a fructose-1-phosphate aldolase-dependent pathway . Experimental strategies to map pathways include:
- Gene knockout studies : Silencing tagK or tagA genes to observe pathway disruption .
- Isotopic tracing : Using 13C-labeled DT1P to track carbon flux in bacterial lysates .
Q. How can researchers resolve contradictions in reported inhibitory effects of DT1P on glycolytic enzymes?
DT1P inhibits fructose phosphate aldolase in E. coli but not in Streptococcus species. To address this:
- Perform enzyme kinetics assays with purified proteins to compare substrate binding affinities (e.g., Km and Vmax) .
- Use molecular docking simulations to analyze structural differences in active sites across species .
- Validate findings with metabolomic profiling to quantify downstream intermediates (e.g., tricarboxylic acid cycle metabolites) .
Q. What experimental designs are optimal for studying DT1P’s role in microbial phosphotransferase systems (PTS)?
- In vitro reconstitution : Combine purified PTS components (e.g., PEP, EI, HPr, and TagK) to measure DT1P synthesis rates under varying ATP/ADP ratios .
- Competitive inhibition assays : Co-incubate DT1P with fructose 1-phosphate to assess substrate specificity of kinases .
- Phosphoproteomics : Identify phosphorylation dynamics in PTS-related proteins using LC-MS/MS .
Q. How can researchers address challenges in quantifying DT1P in complex biological matrices?
- Chromatographic separation : Use anion-exchange HPLC with UV detection (210 nm) to isolate DT1P from co-eluting phosphorylated sugars .
- Enzymatic amplification : Couple DT1P to NADH-linked assays (e.g., via glycerophosphate dehydrogenase) for sensitive fluorometric detection .
- Standard curve validation : Include internal standards (e.g., 13C-DT1P) to correct for matrix effects .
Q. Methodological Notes
Properties
Molecular Formula |
C6H13O9P |
---|---|
Molecular Weight |
260.14 g/mol |
IUPAC Name |
[(3S,4S,5R)-3,4,5,6-tetrahydroxy-2-oxohexyl] dihydrogen phosphate |
InChI |
InChI=1S/C6H13O9P/c7-1-3(8)5(10)6(11)4(9)2-15-16(12,13)14/h3,5-8,10-11H,1-2H2,(H2,12,13,14)/t3-,5+,6-/m1/s1 |
InChI Key |
ZKLLSNQJRLJIGT-PQLUHFTBSA-N |
Isomeric SMILES |
C([C@H]([C@@H]([C@@H](C(=O)COP(=O)(O)O)O)O)O)O |
Canonical SMILES |
C(C(C(C(C(=O)COP(=O)(O)O)O)O)O)O |
physical_description |
Solid |
Origin of Product |
United States |
Disclaimer and Information on In-Vitro Research Products
Please be aware that all articles and product information presented on BenchChem are intended solely for informational purposes. The products available for purchase on BenchChem are specifically designed for in-vitro studies, which are conducted outside of living organisms. In-vitro studies, derived from the Latin term "in glass," involve experiments performed in controlled laboratory settings using cells or tissues. It is important to note that these products are not categorized as medicines or drugs, and they have not received approval from the FDA for the prevention, treatment, or cure of any medical condition, ailment, or disease. We must emphasize that any form of bodily introduction of these products into humans or animals is strictly prohibited by law. It is essential to adhere to these guidelines to ensure compliance with legal and ethical standards in research and experimentation.