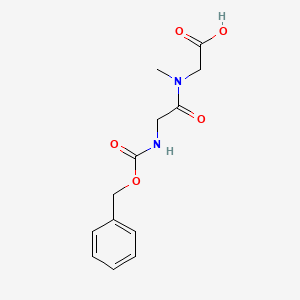
Z-Gly-sar-OH
Overview
Description
Z-Gly-sar-OH, also known as N-[(phenylmethoxy)carbonyl]glycylsarcosine, is a dipeptide derivative. It is composed of glycine and sarcosine, with a benzyloxycarbonyl (Z) protecting group attached to the glycine residue. This compound is often used in peptide synthesis and research due to its stability and reactivity.
Mechanism of Action
Target of Action
The primary target of Z-Gly-Sar-OH is the peptide transporter PEPT-1 (SLC15A1) . This transporter plays a crucial role in the nutritional supply with amino acids by mediating the intestinal influx of dipeptides and tripeptides generated during food digestion . It is also involved in the uptake of small bioactive peptides and various therapeutics .
Mode of Action
This compound, a dipeptide, interacts with its target, PEPT-1, by being recognized and transported by this peptide transporter . The compound comprises an N-methylated peptide bond that increases stability against enzymatic degradation , which is crucial for its interaction with PEPT-1.
Biochemical Pathways
The uptake and transport of this compound in cells are mediated by PEPT-1 . The PI3K/AKT signaling pathway regulates the absorption of small peptides . Inhibition of this pathway can significantly suppress the expression of PEPT-1, thus impairing the uptake and transport of this compound .
Pharmacokinetics
The pharmacokinetics of this compound is closely related to its interaction with PEPT-1. The compound exhibits favorable sensitivity in positive ion mode due to the protonation of the amino terminus of the dipeptide in acidic conditions . .
Result of Action
The result of this compound’s action is the successful transport of the compound into cells via PEPT-1 . This process is crucial for the overall health and productivity of organisms, as it contributes to the nutritional supply with amino acids .
Action Environment
The action of this compound is influenced by several environmental factors. For instance, the uptake and transport of the compound are significantly higher at 37°C than at 4°C . The optimal pH for transport and uptake is 6.0–6.5 . The presence of certain dipeptides can decrease these properties . Furthermore, the compound’s action can be impaired by inhibitors of the PI3K/AKT signaling pathway .
Biochemical Analysis
Cellular Effects
It has been used in studies involving bovine intestinal epithelial cells (BIECs), where it was found that the uptake and transport of Z-Gly-sar-OH were significantly higher at 37°C than at 4°C . The optimal pH for transport and uptake was found to be between 6.0 and 6.5 .
Molecular Mechanism
It is known to be used in proteomics research
Temporal Effects in Laboratory Settings
It has been observed that the uptake and transport of this compound in BIECs are time-dependent .
Transport and Distribution
Preparation Methods
Synthetic Routes and Reaction Conditions
Z-Gly-sar-OH can be synthesized through a series of peptide coupling reactions. One common method involves the reaction of Z-glycine with sarcosine in the presence of a coupling reagent such as dicyclohexylcarbodiimide (DCC) and a catalyst like 4-dimethylaminopyridine (DMAP). The reaction typically occurs in an organic solvent such as dichloromethane at room temperature. The product is then purified through crystallization or chromatography .
Industrial Production Methods
While specific industrial production methods for this compound are not widely documented, the general principles of peptide synthesis apply. Large-scale production would involve automated peptide synthesizers, which can efficiently couple amino acids and protect groups under controlled conditions. The use of high-purity reagents and solvents ensures the consistency and quality of the final product.
Chemical Reactions Analysis
Types of Reactions
Z-Gly-sar-OH undergoes various chemical reactions, including:
Hydrolysis: The compound can be hydrolyzed to release glycine and sarcosine.
Substitution: The benzyloxycarbonyl group can be substituted with other protecting groups or functional groups.
Coupling Reactions: It can participate in further peptide coupling reactions to form longer peptide chains.
Common Reagents and Conditions
Hydrolysis: Acidic or basic conditions can be used to hydrolyze this compound. For example, hydrochloric acid or sodium hydroxide solutions can facilitate the hydrolysis process.
Substitution: Reagents such as trifluoroacetic acid (TFA) can be used to remove the benzyloxycarbonyl group.
Coupling Reactions: Reagents like DCC, DMAP, and N,N’-diisopropylcarbodiimide (DIC) are commonly used in peptide coupling reactions.
Major Products Formed
Hydrolysis: Glycine and sarcosine.
Substitution: Deprotected glycine-sarcosine dipeptide.
Coupling Reactions: Longer peptide chains with this compound as a building block.
Scientific Research Applications
Pharmacological Applications
1.1. PEPT-1 Mediated Transport Studies
Glycyl-sarcosine is primarily utilized to study the proton-coupled peptide transporter PEPT-1 (SLC15A1). Its structural stability due to N-methylation of the peptide bond makes it resistant to enzymatic degradation, allowing for reliable investigations into peptide transport mechanisms. Research has demonstrated that glycyl-sarcosine serves as a substrate for PEPT-1, facilitating the understanding of drug absorption processes in the intestine .
1.2. Inhibition Studies
Glycyl-sarcosine has been employed in various inhibition studies to evaluate the effects of different compounds on its uptake via PEPT-1. For instance, competitive inhibition assays have shown that Gly-Pro, losartan, and valaciclovir significantly affect glycyl-sarcosine uptake, with calculated IC50 values indicating their potency as inhibitors . These findings are crucial for developing drugs that can modulate peptide transport systems.
Analytical Method Development
2.1. Quantification Assays
Recent advancements have led to the development of highly sensitive quantification assays for glycyl-sarcosine using UPLC-MS/MS techniques. These assays allow for the detection of glycyl-sarcosine at concentrations as low as 0.1 ng/mL in cell lysates, which is essential for semi-high-throughput screening of PEPT-1 inhibitors . The methodology includes optimized sample preparation protocols that enhance sensitivity and reduce resource requirements.
Parameter | Value |
---|---|
Lower Limit of Quantification (LLOQ) | 0.1 ng/mL |
Dynamic Range | 0.1 - 1000 ng/mL |
Assay Type | UPLC-MS/MS |
Cellular Uptake Studies
3.1. Caco-2 Cell Model
Glycyl-sarcosine is extensively used in Caco-2 cell models to simulate intestinal absorption processes. The uptake studies conducted on Caco-2 cells have provided insights into how various factors such as pH and concentration influence the transport of peptides across intestinal barriers . The results indicate that glycyl-sarcosine maintains a high affinity for PEPT-1, making it an ideal candidate for studying drug absorption mechanisms.
Case Studies and Findings
4.1. Study on Ion Transport and Nutrient Absorption
A study highlighted the role of enteric endocrine cells in nutrient absorption and ion transport, indirectly linking the importance of substrates like glycyl-sarcosine in maintaining intestinal homeostasis . The loss of enteroendocrine cells resulted in significant imbalances in nutrient absorption, emphasizing the need for effective transport substrates.
4.2. Inhibition Mechanism Exploration
Further investigations into the inhibition mechanisms revealed that various compounds could modulate glycyl-sarcosine uptake through competitive inhibition pathways, providing valuable data for drug design targeting PEPT-1 .
Comparison with Similar Compounds
Similar Compounds
Glycine-sarcosine (Gly-Sar): A simpler dipeptide without the benzyloxycarbonyl protecting group.
N-methylglycine (Sarcosine): A single amino acid derivative.
N,N-dimethylglycine: A dimethylated derivative of glycine.
Uniqueness
Z-Gly-sar-OH is unique due to the presence of the benzyloxycarbonyl protecting group, which provides stability and allows for selective reactions in peptide synthesis. This makes it a valuable tool in the synthesis of complex peptides and proteins .
Biological Activity
Z-Gly-sar-OH, a derivative of glycine, has garnered attention in recent years for its biological activities, particularly in relation to its role as a dipeptide. This article reviews the current understanding of its biological activity, including its mechanisms of action, pharmacokinetics, and potential therapeutic applications.
- Molecular Formula : C₁₁H₁₃NO₄
- Molecular Weight : 223.23 g/mol
- CAS Number : 39608-31-6
This compound functions primarily through its interaction with peptide transporters and various biological pathways:
- Transport Mechanism :
- Inhibition Studies :
Biological Activity
This compound has been linked to various biological activities:
- Neuroprotective Effects : Research indicates that this compound may enhance neuronal survival by modulating reactive oxygen species (ROS) production. Inhibition of prolyl oligopeptidase (POP), an enzyme involved in neurodegenerative processes, has been shown to improve cell viability in neuroblastoma models .
- Anti-inflammatory Properties : The compound has been associated with reduced inflammation through its role in generating immunoactive peptides from larger proteins. This suggests potential therapeutic applications in inflammatory diseases .
Case Studies
Several studies have explored the effects of this compound in various biological contexts:
- Neuroblastoma Cell Lines :
- Gastrointestinal Absorption :
Research Findings Summary
Properties
IUPAC Name |
2-[methyl-[2-(phenylmethoxycarbonylamino)acetyl]amino]acetic acid | |
---|---|---|
Source | PubChem | |
URL | https://pubchem.ncbi.nlm.nih.gov | |
Description | Data deposited in or computed by PubChem | |
InChI |
InChI=1S/C13H16N2O5/c1-15(8-12(17)18)11(16)7-14-13(19)20-9-10-5-3-2-4-6-10/h2-6H,7-9H2,1H3,(H,14,19)(H,17,18) | |
Source | PubChem | |
URL | https://pubchem.ncbi.nlm.nih.gov | |
Description | Data deposited in or computed by PubChem | |
InChI Key |
DNSQLCGFBZSRPW-UHFFFAOYSA-N | |
Source | PubChem | |
URL | https://pubchem.ncbi.nlm.nih.gov | |
Description | Data deposited in or computed by PubChem | |
Canonical SMILES |
CN(CC(=O)O)C(=O)CNC(=O)OCC1=CC=CC=C1 | |
Source | PubChem | |
URL | https://pubchem.ncbi.nlm.nih.gov | |
Description | Data deposited in or computed by PubChem | |
Molecular Formula |
C13H16N2O5 | |
Source | PubChem | |
URL | https://pubchem.ncbi.nlm.nih.gov | |
Description | Data deposited in or computed by PubChem | |
DSSTOX Substance ID |
DTXSID20293391 | |
Record name | Z-GLY-SAR-OH | |
Source | EPA DSSTox | |
URL | https://comptox.epa.gov/dashboard/DTXSID20293391 | |
Description | DSSTox provides a high quality public chemistry resource for supporting improved predictive toxicology. | |
Molecular Weight |
280.28 g/mol | |
Source | PubChem | |
URL | https://pubchem.ncbi.nlm.nih.gov | |
Description | Data deposited in or computed by PubChem | |
CAS No. |
7801-91-4 | |
Record name | 7801-91-4 | |
Source | DTP/NCI | |
URL | https://dtp.cancer.gov/dtpstandard/servlet/dwindex?searchtype=NSC&outputformat=html&searchlist=89181 | |
Description | The NCI Development Therapeutics Program (DTP) provides services and resources to the academic and private-sector research communities worldwide to facilitate the discovery and development of new cancer therapeutic agents. | |
Explanation | Unless otherwise indicated, all text within NCI products is free of copyright and may be reused without our permission. Credit the National Cancer Institute as the source. | |
Record name | Z-GLY-SAR-OH | |
Source | EPA DSSTox | |
URL | https://comptox.epa.gov/dashboard/DTXSID20293391 | |
Description | DSSTox provides a high quality public chemistry resource for supporting improved predictive toxicology. | |
Retrosynthesis Analysis
AI-Powered Synthesis Planning: Our tool employs the Template_relevance Pistachio, Template_relevance Bkms_metabolic, Template_relevance Pistachio_ringbreaker, Template_relevance Reaxys, Template_relevance Reaxys_biocatalysis model, leveraging a vast database of chemical reactions to predict feasible synthetic routes.
One-Step Synthesis Focus: Specifically designed for one-step synthesis, it provides concise and direct routes for your target compounds, streamlining the synthesis process.
Accurate Predictions: Utilizing the extensive PISTACHIO, BKMS_METABOLIC, PISTACHIO_RINGBREAKER, REAXYS, REAXYS_BIOCATALYSIS database, our tool offers high-accuracy predictions, reflecting the latest in chemical research and data.
Strategy Settings
Precursor scoring | Relevance Heuristic |
---|---|
Min. plausibility | 0.01 |
Model | Template_relevance |
Template Set | Pistachio/Bkms_metabolic/Pistachio_ringbreaker/Reaxys/Reaxys_biocatalysis |
Top-N result to add to graph | 6 |
Feasible Synthetic Routes
Disclaimer and Information on In-Vitro Research Products
Please be aware that all articles and product information presented on BenchChem are intended solely for informational purposes. The products available for purchase on BenchChem are specifically designed for in-vitro studies, which are conducted outside of living organisms. In-vitro studies, derived from the Latin term "in glass," involve experiments performed in controlled laboratory settings using cells or tissues. It is important to note that these products are not categorized as medicines or drugs, and they have not received approval from the FDA for the prevention, treatment, or cure of any medical condition, ailment, or disease. We must emphasize that any form of bodily introduction of these products into humans or animals is strictly prohibited by law. It is essential to adhere to these guidelines to ensure compliance with legal and ethical standards in research and experimentation.